Deep in the bellies of the great pyramids, pharaohs and queens began their voyage into the afterlife. Salt-cured fish and fowl were among the many provisions entombed with Egyptian royalty to nourish them on their long journey. Egyptians have been using salt to extend the lifetime of food for at least 4,000 years. Indeed, for most of recorded human history, salt curing has been a preferred way to preserve food, a necessity for cold winters, distant wars, or long expeditions to new worlds—from the Americas to the afterlife.
“The big change comes in the 19th century,” says food historian Harvey Levenstein. In 1810 Napoleon Bonaparte awarded French innovator Nicolas Appert 12,000 francs for his invention of canning. Napoleon had an army to feed and needed a ready supply of long-lasting food. Later that same century industrialists used Appert’s discovery and the inventions of many others to begin mass-producing canned produce, cereal, and crackers. For the first time food was made at factories far from consumers. And by the 1910s the food we eat was well on its way to industrialization.
What follows are three food-processing stories each with its own defining moment in the 1910s.
We start with the American canning industry, which in 1913 launched a research center to study the bacteriology of food spoilage. The reason? A public terrified of being poisoned by canned foods.
Across the Atlantic, in 1912 French chemist Louis Camille Maillard discovered that many of the flavors, odors, and colors that make food inviting are the result of heating together amino acids and sugars. Think of the delicious odors wafting off freshly baked bread, the rich color of soy sauce, or the delectable flavor of a barbecued steak. The Maillard reaction became one of food science’s most important phenomena. Unfortunately for food lovers scientists later discovered a dark side to this reaction.
Finally, the late 1910s saw the launch of an industrialized fresh-food icon: iceberg lettuce. Lettuce tycoons used California’s endless growing season, state-of-the-art refrigeration technology, and an extensive rail system to make iceberg one of America’s most consumed vegetables. Chosen for its travel hardiness rather than aesthetic qualities, iceberg lettuce would set the precedent for the modern fresh-produce industry.
Canning: From Craft to Science
Canning has its roots in France, perhaps surprising given that French cuisine is associated with dishes made from garden-fresh ingredients. But in 1795 the French government faced the fact that it’s not easy winning battles abroad when your soldiers are hungry and malnourished. That year the French government established a 12,000-franc prize for whoever could find a way to preserve food—from milk and meat to fruits and vegetables.
Nicolas Appert took more than a decade to develop a canning process that involved cooking food in glass jars and then sealing them with cork. In 1810 Appert published L’art de conserver pendant plusiers années toutes les substances animales et végétales and collected his prize money. Appert’s achievement didn’t escape the notice of British inventor Peter Durand, who soon applied for a British patent to preserve food using techniques similar to Appert’s, though Durand sealed his food in tin containers as well as glass jars.
Canning was initially used to keep soldiers and explorers fed, yet nobody knew why canning worked. Even after the establishment of germ theory in the late 19th century, canners couldn’t figure out exactly why some of their food turned rotten. In the early 20th century, notes food historian Gabriella M. Petrick, newspapers were full of accounts of sorority picnics gone awry from salads made from canned vegetables or children near death from botulism. For that reason many looked upon canned food—already in existence for a century—with suspicion and fear.
Canning was initially used to keep soldiers and explorers fed, yet nobody knew why canning worked.
The Heinz Company realized bad cans were bad for business. From the 1890s to 1920s the company mandated weekly manicures for its canning-factory workers to ensure that any bacteria lurking beneath employees’ fingernails stayed out of the food. Heinz employees were required to shower and change their underwear regularly.
“This forced regime sounds a bit draconian,” Petrick says, but in an era when food-factory hygiene was not universally adopted, what might have been intrusive to workers was good for consumers.
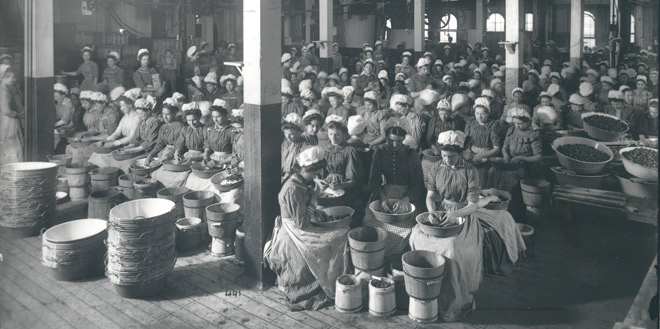
Heinz factory workers cleaning strawberries before canning, ca. 1904. To ensure the quality of its products, Heinz required workers to clean their nails weekly and shower regularly.
Some canning companies killed microbes with additives, such as sodium benzoate (which is still used today in canned food) and formaldehydes (now known to be carcinogenic), Levenstein says. According to Petrick, some of the larger canning companies, such as Heinz, Libby, and Campbell’s, installed expensive steam-retort technology developed in the 1870s. Steam retorts worked like steam engines, using pressure to raise canning temperatures high enough to kill pathogenic microbes. Smaller canning outfits relied on open kettles and had less consistent results. Yet some batches of cans, even when made with the era’s most advanced techniques, would explode after months on the shelf; the contents of other cans might mysteriously sour.
American canning companies eventually banded together to discover the scientific underpinnings of what was still mostly a craft, Petrick says. In 1913 they launched an industry-wide research agency, the Research Laboratory of the National Canners Association, to study the elusive bacteriology responsible for all manner of spoiled canned food. The research done by the agency in the decades that followed helped resolve the problem of spoilage and led to improved flavor, color, nutritional value, and texture.
Before this time canners had discovered through trial and error that the best way to avoid bad cans was to cook food at high temperatures for long periods, an approach that sacrificed flavor. “Food safety and palatability were often at cross-purposes,” Petrick remarks. One technique employed was adding salt to the vegetables. For string beans long cooking times leach out the green color, creating an unappealing grayish-toned bean, says Roger Clemens, a nutrition and food-safety expert. “By adding [salt] during processing, we could reduce cooking time and maintain the color.” (Yet what was once a desirable additive has become a modern health culprit, guilty of contributing to the rise in hypertension, heart disease, and obesity seen in many Western societies.)
One of the major canning innovations of the 20th century was the development of aseptic processing, notes food scientist John Floros. This technique, which involves sterilizing the container separately from the food, was invented in Switzerland in the 1960s for processing milk but is now used widely for canned tomatoes and many juices.
Small volumes of food are heated to a precise temperature and for a precise time in order to avoid overcooking and loss of nutrients, explains food scientist Philip Nelson, who won the 2007 World Food Prize for his research on making aseptic processing workable on an industrial scale. “When you put something in a can, you have to heat to the center, so you end up overheating what’s on walls,” says Nelson. Aseptic processing helps avoid this problem. “Then by storing [the food] in an oxygen-free environment you prevent reactions that affect flavor, color, and nutrients.”
In more recent decades the food industry has looked into alternative methods of making their products safe, including gamma irradiation, which preserves well but creates what Nelson describes as “wet dog–like flavors.” More successful, Floros says, is the use of pressure to preserve food. Fresh guacamole sold in supermarkets is pressure-processed to keep it from turning brown. Prepackaged raw oysters are also preserved with pressure.
Despite all the 20th-century alternatives, Appert’s technology—the can—still reigns supreme for long-term storage.
Uncovering the Mysteries of the Maillard Reaction
In 1997 construction workers digging a railroad tunnel in southwestern Sweden began to feel numb. Cows in the vicinity weren’t faring well either; some became paralyzed and eventually died. Fish in nearby waters floated to the surface.
Authorities discovered that acrylamide, a component in some of the sealant used in the tunnel and a potent carcinogen in rats, was to blame. Swedish toxicologist Margareta Törnqvis and her doctoral student Eden Tareke were called in to evaluate the exposure of tunnel workers to the dangerous substance. Their research unexpectedly led them to the kitchen and to the discovery that a cherished chemical reaction in food science, the Maillard reaction, also puts acrylamide in our dinner. The publication of these results in 2002 launched a decade-long transformation of the food industry as researchers tried to figure out how to avoid producing the poison.
Normally the Maillard reaction is described with appetizing adjectives, and rightly so. In 1912 French chemist Louis-Camille Maillard published a paper that describes what happens when amino acids react with sugars at elevated temperatures: the creation of many delightful flavors and odors, such as the smell of popcorn and the taste and flavor of roasted coffee. The Maillard reaction is also responsible for the brown of barbecued steak, baked bread, and soy sauce. (Maillard reactions differ from but are often mistaken for caramelization, which is a browning of sugars exposed to heat.)
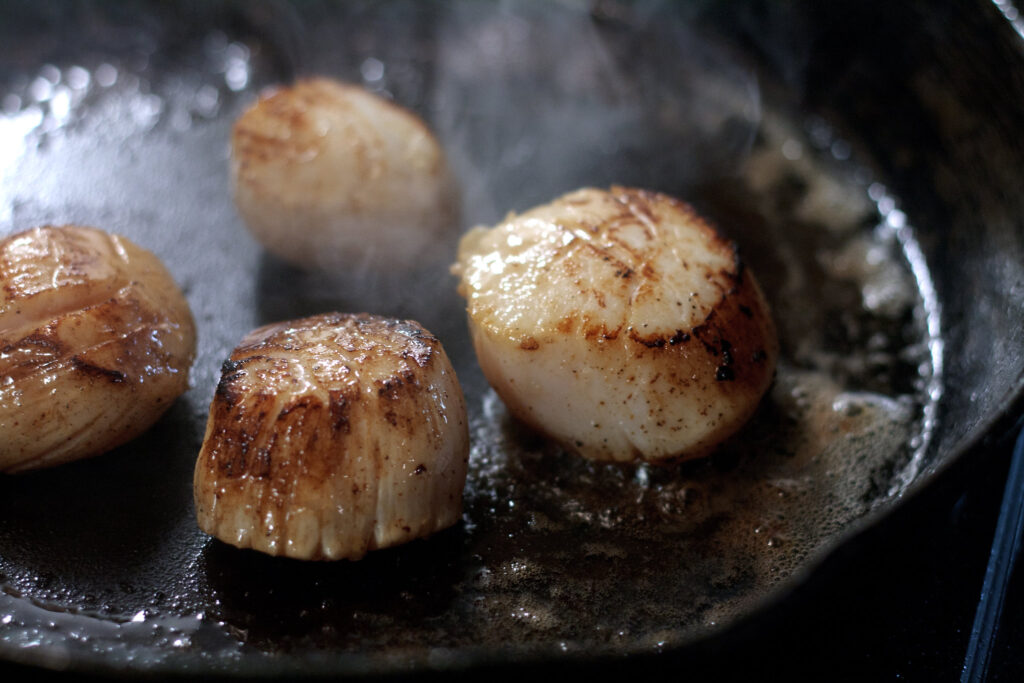
In 1912 Louis Maillard was the first to describe the chemical reactions in grilled, sautéed, and baked foods that make them so delicious and, we know now, a little unhealthy.
“There wasn’t much of what you could call flavor chemistry before Maillard,” remarks historian Alan Rocke. “In the 1800s the German chemist Justus von Liebig published ideas about the importance of protein extracts of beef, and a lawyer, Jean Anthelme Brillat-Savarin, published heavily cited anecdotal ponderings on taste, but Maillard was the first to tackle serious food chemistry.”
The Maillard reaction proceeds through three thorny, intricate steps, which can each produce hundreds of different products. In addition, most foods have many different kinds of amino acids and sugars, creating a cornucopia of possible participants in the reaction.
Not until 1953 did the chemistry community get a handle on how all these flavor compounds could be produced, says food chemist Vincenzo Fogliano. That year a chemist at the U.S. Department of Agriculture, John E. Hodge, established a mechanism for the Maillard reaction. “Maillard discovered the reaction, but Hodge understood it,” Fogliano says.
Normally the Maillard reaction is described with appetizing adjectives, and rightly so.
Fortuitously, developments in gas chromatography and protein mass spectrometry that same decade permitted food scientists to measure Maillard products in food, notes Floros. From that point the food industry had the tools to control the chemistry of cooking amino acids and sugars, both to orchestrate the production of pleasing flavors and odors and to avoid the offensive ones. However, this task is complicated by the fact that the Maillard reaction can produce thousands of different molecules with even slight changes to temperature, moisture levels, or pH, says food chemist Thomas Hofmann.
Sometimes a Maillard product will be universally pleasant, such as the 2,3-butanedione found in popcorn and grilled steak. Other times a product that is desirable in some dishes is less welcome in others, Hofmann explains. For example, the compound 2-acetyl-1-pyrroline gives crusty bread and basmati rice a pleasant odor and flavor but produces a strange aftertaste when found in ultra-high-temperature pasteurized milk. Maillard reactions can also change the texture and consistency of proteins in food, making yogurt more gelatinous or cheese softer and creamier, says food chemist Thomas Henle. Then there are the negative products, such as the loss of vitamin C and B1 in Maillard reactions during cooking, states food scientist Cathy Davies. And there’s the production of acrylamide.
When Tareke and Törnqvis, the toxicologists in Sweden, were asked to examine the exposure of the sick construction workers to acrylamide, they did what any reputable scientists would do: they compared the levels of acrylamide in the sick workers to those in the general population. To their surprise they found unexpectedly high levels of acrylamide in the control group. Tareke was also simultaneously comparing acrylamide levels in wild animals and domesticated pets for her doctoral work, and to her further surprise found high levels in pets.
Given that a major difference between wild and domesticated animals is the amount of processed food they consume, Törnqvis and Tareke suspected that the acrylamide in the human controls might be attributable to their eating highly processed food. In 2002 they showed that processed food, especially potato chips but also common baked bread, did in fact contain acrylamide. Facing public outcry, food-industry associations banded together to fund research on exactly how the Maillard reaction led to acrylamide production and how it might be thwarted. One of the most promising techniques developed for acrylamide prevention is using an enzyme called asparaginase to break down the amino acid asparagine, says food scientist Monica Anese. Because acrylamide is created when asparagine reacts with sugar, removing the amino acid at the outset decreases acrylamide levels in the final foodstuff. Another strategy, she says, is to lower cooking temperature since acrylamide is produced under high heat. The downside is less browning of cookies and bread, an unpopular option with consumers.
For Real?
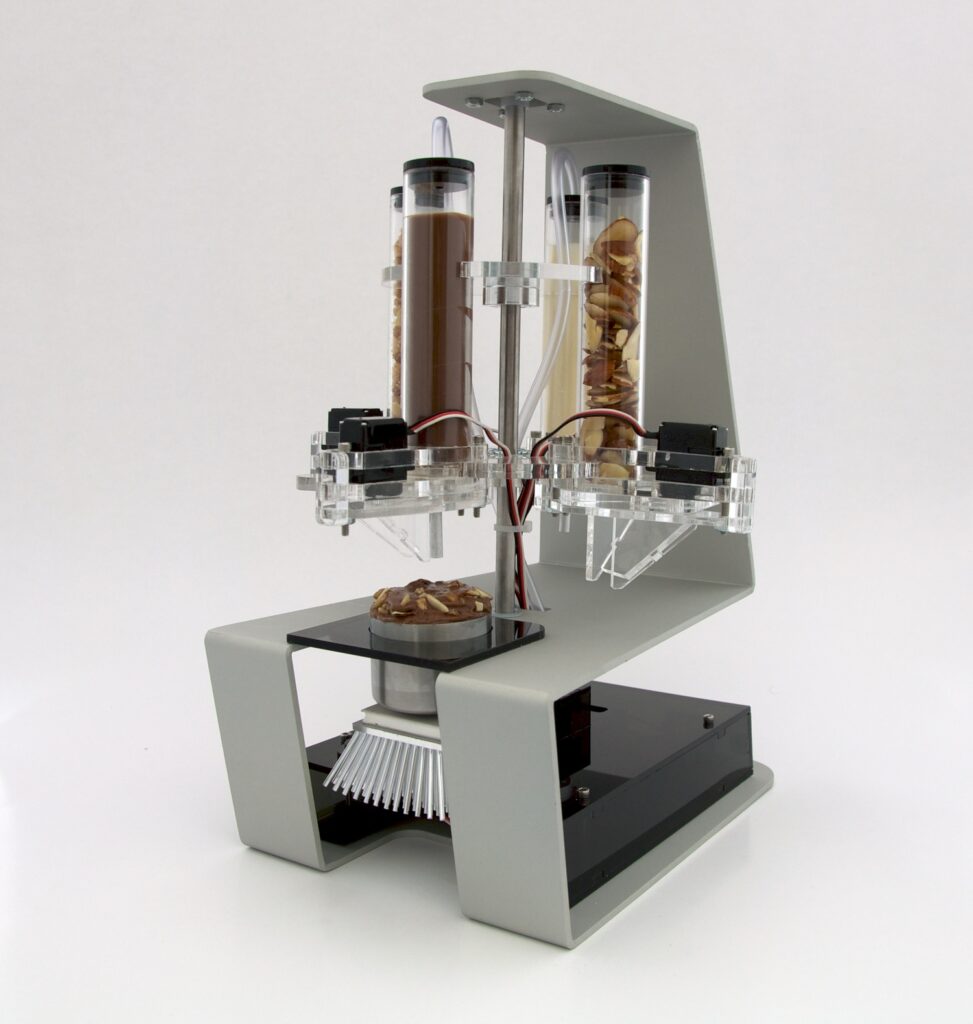
A digital chocolatier designed by MIT’s Marcelo Coelho.
It is tempting to wonder how the food industry will evolve. If you ask Hervé This, a food chemist and a cocreator of molecular cuisine, he’ll describe his new passion: note-by-note cuisine. Note-by-note adherents build food from chemical scratch by identifying the specific molecules in a dish responsible for flavor and texture. (This author tasted a note-by-note fish custard and an orange cocktail, and so far she declines to give her full approval.)
Hervé This argues that future energy concerns may make shipping fresh produce around the world, or even just across the country, prohibitively expensive. Likewise, energy costs may make restaurant standards, such as reduction sauces that are slowly heated for hours, financially impossible. With a mind toward sustainability This wonders whether agricultural waste can be transformed into the sensory molecules used to build note-by-note food.
To many, This’s future is a dystopian one. But he’s not the only person to propose curious constructions of food. Inventor Pablos Holman told NPR’s Freakonomics in 2011 that he’s trying to build a 3-D printer for food. He imagines dried food stored in toner cartridges that could be deposited pixel by pixel, laser cooked pixel by pixel, and thus built into a meal.
If all this seems ridiculous, just consider what canning inventor Nicolas Appert would have thought of microwave dinners.
Almost Garden Fresh
Americans may occasionally wax philosophical about the flavorful delights of garden-ripe produce, but as a population we’re more willing to sacrifice taste and aroma for year-round access to fresh fruits and vegetables.
The now century-old industrialization of produce, particularly fresh lettuce and tomatoes, has led to the growing of varieties that can survive long-distance transport. While our produce looks good with jetlag, it often comes at the cost of flavor and aroma. But there is some good news: in some cases a new understanding of the biochemistry of ripening and decay may help the agriculture industry give us the best of both worlds.
During the late 1910s and early 1920s iceberg lettuce went from near obscurity to one of America’s most eaten fresh vegetables. It was the only variety of lettuce that could survive a train trip from California fields to East Coast markets without turning into a sodden mess of goop, explains food historian Petrick. Before World War I most lettuce consumed on the East Coast was leaf or butter lettuce, and it was eaten only during the spring and summer growing seasons, she says.
Petrick found that in the later part of the 1910s California farmers began to capitalize on their year-round growing season, excellent rail infrastructure (built to transport sugar beets cross-country), and better train-car refrigeration. Iceberg lettuce was the chosen food not for its flavor—it has little—but for its long shelf life and resiliency. Iceberg’s ideal storage temperature was just a degree or so above freezing, temperatures that would make other lettuces limp, Petrick says. Initially crushed ice was used to keep the lettuce cool, but in the 1950s vacuum-cooling technology originally developed during World War II reduced spoilage across long distances.
The changeover was quick. In 1917, 50% more butter lettuce was being shipped throughout the United States than iceberg. By 1925 four times more iceberg lettuce was shipped than butter lettuce, Petrick has discovered. “Instead of having truly high-quality, fresh-from-the-field lettuce, Americans ate lettuce that was ‘good enough’ to put on the table every day,” she says.
As refrigerators became commonplace in the late 1920s and early 1930s, the food industry, led by entrepreneur Clarence Birdseye, began to experiment with freezing rather than canning fresh fish and produce. It wasn’t a roaring success at first, notes the food historian Levenstein. Both the frozen produce and the wax paper that wrapped it would melt into mush. The wax paper was eventually substituted for newly available plastic, and Birdseye rebranded frozen food as “frosted food,” which didn’t melt but “defrosted.” It was a clever marketing trick, says Levenstein, because it made everything from frozen peas to berries sound more appealing. Birdseye also developed flash freezing in the 1920s, which preserves food texture. In slow freezing ice crystals form in cell walls and break them down, making the thawed food mushy.
Birdseye rebranded frozen food as “frosted food,” which didn’t melt but “defrosted.”
Another strategy widely used today is to delay ripening itself: pick produce early, transport it to market, ignite ripening, and sell. Unripe produce is usually hardier and can better withstand the jostling and other insults that take place during global transport, remarks plant biochemist Harry Klee. In the best-case scenario ripening can be timed to coincide with the produce’s appearance on store shelves. “But all this comes at the cost of flavor, color, and texture,” he adds.
Since ripening is a fruit’s equivalent of hitting puberty, controlling the process is a matter of controlling a plant’s ripening hormones and the genes these hormones activate. In the case of the common supermarket tomato, breeders have selected for a plant with a genetic mutation that interferes with the softening that occurs during ripening, says Klee. Unfortunately the same mutation prevents the tomato from producing the flavor compounds that are part of the ripening. No matter how long you leave a standard supermarket tomato in the sun, it will never achieve the flavor and texture of an heirloom variety.
Although this type of tomato has been on supermarket shelves for decades, not until 2012 did a team of researchers at Cornell University figure out the reason for its flavor failure: in short, a mutation in a gene called SlGLK2. The gene’s corresponding and dysfunctional protein won’t activate the production of chloroplasts, which harness light energy to produce sugar. Chloroplasts are also where many of the tomato’s characteristic flavor and odor compounds are produced.
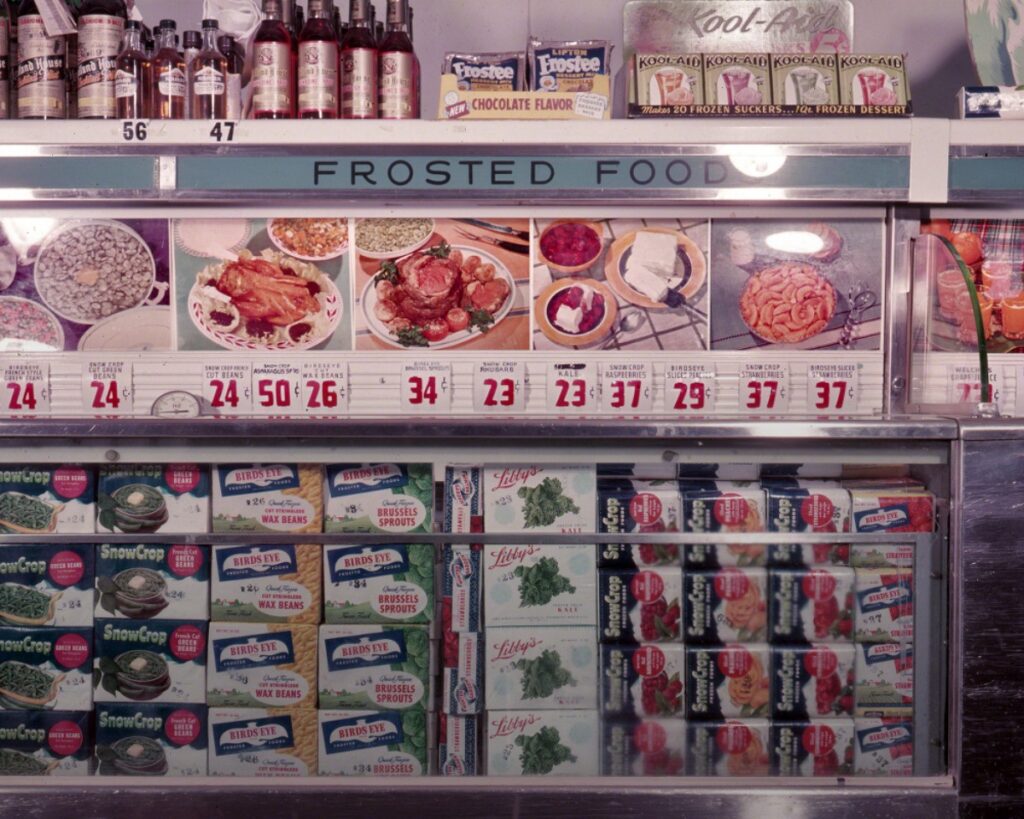
By the late 1920s frozen vegetables had entered the consumer food market. Birdseye developed flash freezing to preserve the texture and flavor of frozen foods.
In addition to breeding delayed ripening into plants, the produce industry also employs chemistry to achieve the same end. For example, the ripening of many fruits, including bananas, tomatoes, apples, melons, apricots, and papayas can be triggered by ethylene, a gaseous plant hormone. Ethylene is what turns bananas from green to yellow and tomatoes from green to red. When fruits sense ethylene, a chain reaction begins: the fruit ripens and produces its own ethylene, which is then sensed by and activates the ripening of nearby fruit.
Ethylene-sensitive fruits are picked green and shipped in closed containers that also hold chemicals, such as potassium permanganate, that can absorb or disable ethylene. Another strategy, approved in the United States and the European Union, is to douse unripe fruit with 1-methylcyclopropene. This molecule blocks the ethylene receptor in many fruits for up to 12 days so that even if some of the ripening hormone is wafting about, the fruits can’t detect it.
Last but not least, pairing vegetables with the proper packaging can maintain freshness. Even off the vine, fruits and vegetables continue to respire, taking in oxygen and releasing carbon dioxide. Put vegetables in a closed environment where no respiration is possible, and they deviate into a different metabolic strategy, one that destroys tissue, thereby producing muck in a bag. To counter this, says Floros, the produce industry has developed plastic packaging that is slightly permeable to oxygen and carbon dioxide, creating a microenvironment that slows down respiration and decay.
In the last century the food industry has figured out how to tweak plant biology in order to control puberty and delay aging. Most of this tinkering was done by trial and error without detailed knowledge of what was happening on a molecular level in plants and their fruits. Over the past decade researchers have sequenced the genome of a variety of plants, including the tomato, and they’ve figured out the three-dimensional structures of many of the receptors in plants that detect ripening hormones. This knowledge is allowing plant biologists to figure out, in retrospect, how some of their tinkering has interfered with ripening and led to flavor loss—a loss that most consumers accepted in exchange for the convenience of out-of-season vegetables. Yet consumers are now increasingly demanding it all: fresh fruit and veggies year-round that truly do taste vine ripe, even if they are not. If the food industry can figure out a way to give people what they want, it will require a lot more tinkering with plant biology, certainly more chemical interventions, and possibly some genetic engineering. Whether future consumers would buy into the outcome is anyone’s guess.