On the morning of June 6, 1822, a young French Canadian man, standing in the American Fur Company store on Mackinac Island in what is now Michigan, fell victim to a grisly accident.
Alexis St. Martin was in his 20s and strong, about 5 feet, 5 inches tall, with dark hair and dark eyes. He worked as a voyageur, a hardy and adventurous job that entailed paddling pelt-laden canoes from Native American villages to the company’s trading posts. As he stood in line that morning to make some trades, someone’s musket accidentally went off, shooting St. Martin in the left side of his chest from about 3 feet away. It was such close range that St. Martin’s shirt caught fire as he fell over. He was presumed dead.
The other traders sent for William Beaumont, the doctor at a U.S. Army fort on the island, who arrived just as St. Martin was being put on a cot. Beaumont found that the shot had ripped through several ribs and lacerated St. Martin’s diaphragm and lower left lung before exiting his body. Most important—at least for our callous purposes—the blast had left a hole in his stomach.
“[I] found a portion of the lung as large as a turkey’s egg, protruding through the external wound, lacerated and burnt,” Beaumont later wrote, “and immediately below this, another protrusion, which, on further examination, proved to be a portion of the stomach, lacerated through all its coats, and pouring out the food he had taken for his breakfast, through an orifice big enough to admit the forefinger.” He doubted St. Martin would survive for 36 hours.
But St. Martin didn’t die. Instead, as the weeks progressed and Beaumont continued to watch over him, his body went through a remarkable healing process, expelling several fragments of cartilage and rib, enduring a violent fever and a fetid wound. Since any food or drink that St. Martin consumed leaked right back out through the hole in his stomach, Beaumont sustained him “by means of nutritious injections per anum” until the doctor was able to apply compresses and adhesive straps around the wound tightly enough to keep food from escaping.
Eventually, St. Martin’s body completely recovered, except for one strange thing: the hole in his stomach refused to close. Instead of binding to themselves, the walls of his stomach adhered to the skin of his abdomen, creating a permanent hole beneath his ribs that led directly into the organ. Beaumont proposed closing the wound (known more formally as a gastric fistula) with stitches, but St. Martin, exhausted by what he had already endured, refused. After months of using bandages to retain food, his stomach eventually solved the problem on its own: it grew an interior valve-like flap of skin that prevented food from escaping but was “easily depressed by the finger,” allowing Beaumont access to what was inside.
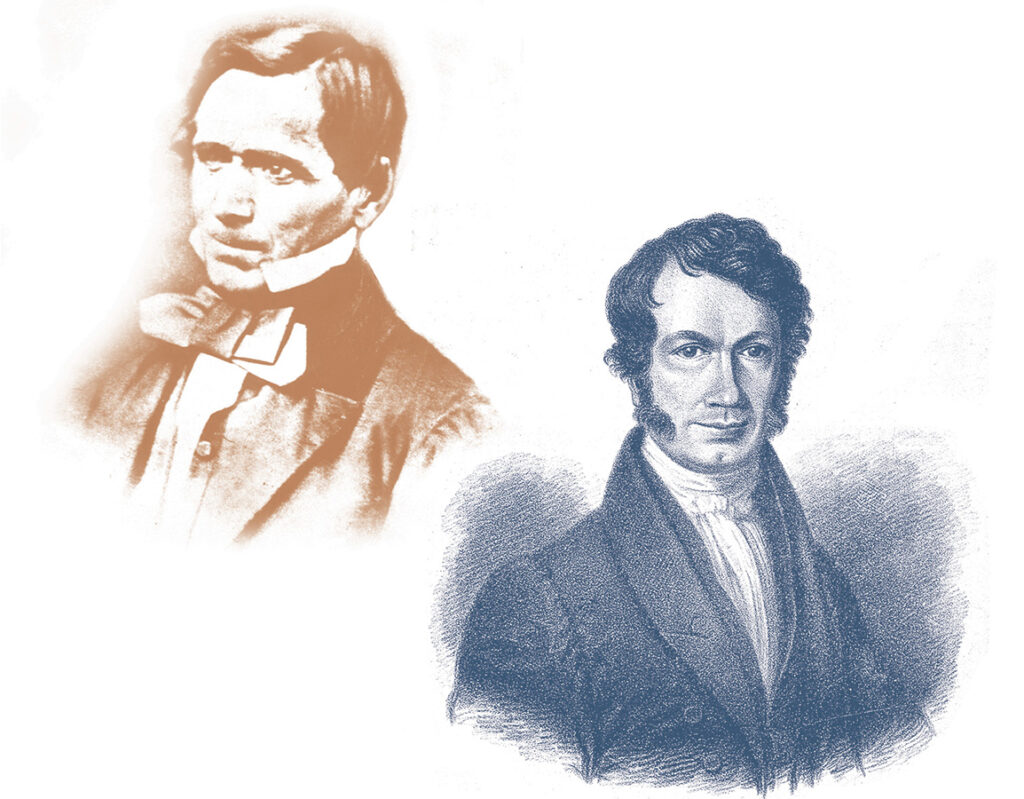
While appalling, the on-again, off-again relationship between Canadian fur trader Alexis St. Martin (left) and physician William Beaumont (right) gave scientists a better understanding of the digestive system’s functions and produced a landmark medical text.
Beaumont quickly realized the experimental potential of his unique patient, who having lost his job as a voyageur was now completely dependent on the doctor.
“When he lies on the opposite side I can look directly into the cavity of the Stomach and almost see the process of digestion,” wrote Beaumont with excitement. “I can pour in water with a funnel, or put in food with a spoon, and draw them out again with a syphon. I have frequently suspended flesh, raw and wasted, and other substances into the perforation to ascertain the length of time required to digest each; and at one time used a tent of raw beef, instead of lint, to stop the orifice, and found that in less than five hours it was completely digested off, as smooth and even as if it had been cut with a knife.”
Thus began the odd relationship between William Beaumont and Alexis St. Martin, an accidental and often complicated partnership that revolutionized our understanding of human digestion.
For centuries physicians thought of food as fuel for an internal heat that “cooked” food in the stomach and intestines. Some thought digestion was caused by fermentation; others, by putrefaction. In the 17th and 18th centuries in particular, a debate had emerged in Europe over what digestive juice was and whether digestion was a chemical or a mechanical process. Adding to the confusion was the enduring popularity of a theory called vitalism, which assumed life was caused by a “vital,” or spiritual, force and that something as miraculous as digestion couldn’t be explained in mere mechanical or chemical terms. These debates carried on into the 19th century, when Beaumont began his prodding.
Beaumont started his first official experiment at midday on August 1, 1825. (By that point St. Martin was living in Beaumont’s home and working as a servant.) A common theory held that the stomach digested each type of food sequentially and indigestion was therefore the result of eating too many different foods at one meal. Beaumont wanted to figure out whether this were true.
An hour later Beaumont pulled out the string and found the cabbage and bread about half digested, but the meat unchanged. He returned them to the stomach. By 2:00 p.m. the à la mode beef was partially digested and the raw beef slightly macerated on the surface. Beaumont reported that the smell and taste of the fluids of the stomach were “slightly rancid” and that “the boy complained of some pain and uneasiness in the breast.” He put the samples back in the stomach. By the time he pulled them out again, St. Martin was “complaining of considerable distress and uneasiness at the stomach” with some pain in his head. The remaining pieces of food were in basically the same condition as before, with the fluid more rancid and sharp. “The boy still complaining,” wrote Beaumont, “I did not return them any more.”
Having participated in several clinical trials myself, I can tell you this is no longer how things work. These days you sign pages and pages of legal releases, spend a day watching television in a hospital room, are monitored by a nurse, and often are reimbursed for your travel expenses.
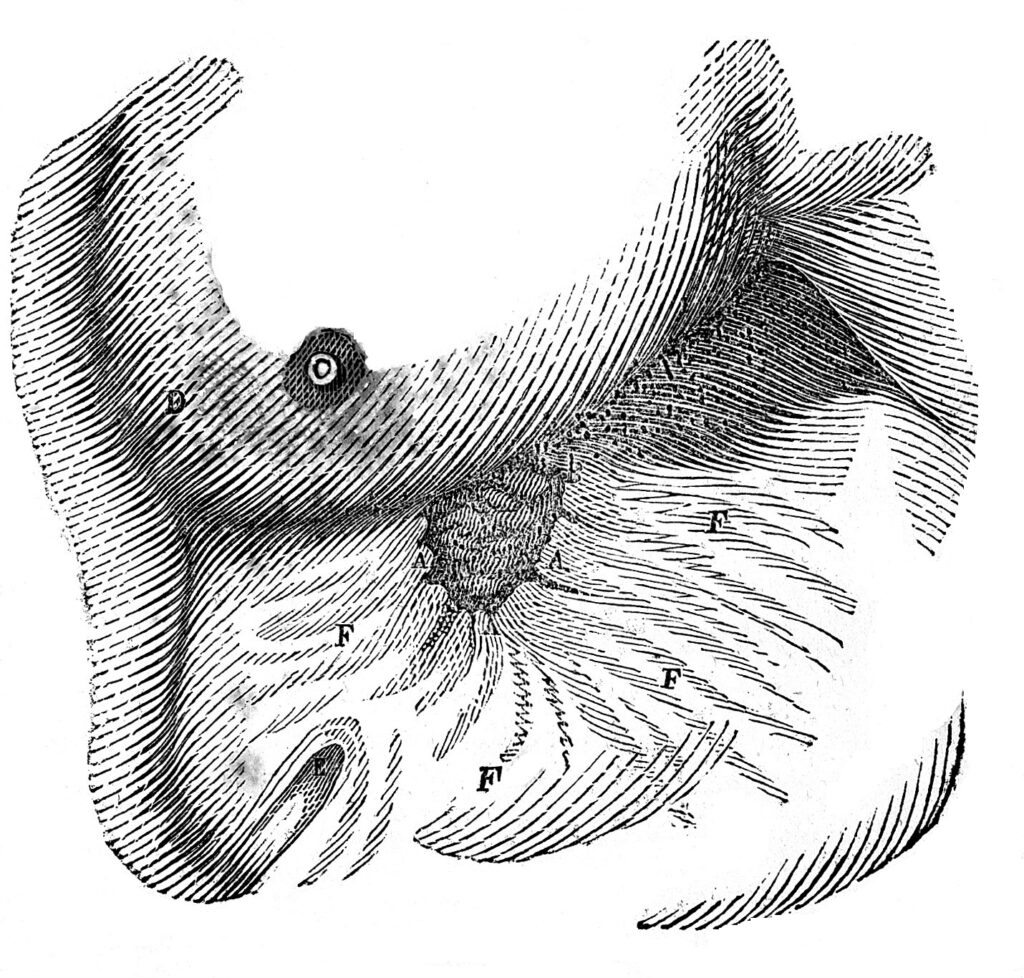
Alexis St. Martin’s fistula.
St. Martin, however, endured 17-hour fasts and constant poking and prodding at the hands of Beaumont. He was made to carry vials of his stomach contents tucked into his armpits to help Beaumont learn about the effect of body heat on digestion. He was called nicknames, such as “Fistulous Alexis” and the “Man with a Lid on His Stomach.” What’s more, “in the intervals of experimenting, he performed all the duties of a common servant,” wrote Beaumont, “chopping wood, carrying burthens [sic], &c., with little or no suffering or inconvenience from his wound.”
Nonetheless, since an illiterate voyageur with a perforated stomach has only so many career opportunities, St. Martin continued to endure the intrusions: he traveled with Beaumont to Fort Niagara, New York, followed by trips to Burlington, Vermont, and Plattsburgh, New York, the experiments continuing all the while. But in Plattsburgh the proximity of his native Canada proved too much of a temptation to resist. “From the latter place he returned to Canada, his native place,” wrote Beaumont, “without obtaining my consent.”
About two years later Beaumont tracked St. Martin down with the help of a fur-trading friend who reported that St. Martin (“your ungrateful boy”) was married and “poor and miserable beyond description.” It took two years more, but Beaumont eventually convinced St. Martin to return to him and continue the experiments.
The men joined and parted several times, St. Martin repeatedly retreating to the peace of Canada but driven by poverty to return to Beaumont’s side. He eventually died in 1880, outliving Beaumont—who was felled by an icy set of stairs—by about 28 years.
Several researchers hoped to perform an autopsy on St. Martin’s corpse, and there were even plans to preserve his stomach in the Army Medical Museum—but his family refused. They were so insistent that his body not be used for another scientific experiment they allowed it to decompose at home to the point that it couldn’t be brought into the church for his funeral service. They then buried it eight feet deep to thwart would-be grave robbers. Enough was enough.
All told, Beaumont performed some 200 experiments on St. Martin over about 10 years, and his 1833 official account, Experiments and Observations of the Gastric Juice and Physiology of Digestion, is considered one of the greatest (and, to my mind, weirdest) surgical texts of pre–Civil War America.
The men’s tortured relationship aside, Beaumont’s observations were revolutionary. Beaumont recorded 51 conclusions about digestion, including that vegetables digested more slowly than meat, that milk coagulates early in digestion, that a healthy stomach’s lining is mucus coated and pale pink, and that the stomach churns its contents to help digestion. His most notable conclusions, however, had to do with gastric juice, a powerful and wondrous substance he examined through as many senses as possible:
Gastric juice analysis might not sound exciting, but at the time, this was cutting-edge stuff. Before the 1820s gastric juice (like digestion itself) was a mystery, with several contradictory theories as to what it was and what it was for. Some thought it was a solvent, others that it was nothing more than glorified saliva. In 1824, shortly before Beaumont’s first experiments on St. Martin, a British physician-turned-chemist named William Prout discovered that the gastric juice of animals contained hydrochloric acid, a substance so strong his peers had difficulty believing it could exist in our bodies without causing harm. Beaumont not only definitively confirmed Prout’s discovery but also found the stomach lining excreted the caustic juice.
If you spend eight hours a day researching the history of nutrition, you will find there are certain illustrations that pop up again and again, as if all textbook editors have access to the same archive of clip art. Often these are straightforward portraits, but occasionally something appears that is weird enough to be memorable. Among the latter is a drawing of an Italian Renaissance physiologist and physician named Santorio Santorio.
Strong jawed and noble in expression, Santorio—also known as Sanctorius—is shown in front of a cloth-covered table, neatly set with cutlery, a wine glass, and several plates of food. He is sitting with perfect posture, back straight, his gaze firm. But he’s not in a normal chair. Instead, he’s perched on the seat of a cage-like contraption dangling from the ceiling from one end of a giant scale.
Santorio’s “weighing chair” was designed to figure out why what he ate and drank weighed more than did his subsequent excretions. This question consumed Santorio; he was obsessed with where the extra weight had gone. He weighed himself before and after all sorts of situations where no urination or defecation had taken place, during exercise or sex. He weighed his food. He weighed his urine. He weighed his feces. Finally, after doing this for some 30 years he came up with the theory of “insensible perspiration”—that some substance was being lost through the skin or the mouth that accounted for the missing weight.
He was onto something: we now know that his weight loss was indeed the result of water lost through his breath and skin, and this water was a by-product of his body’s breaking down of food. But Santorio, unfortunately, would never learn the full explanation. The answer to his question required an understanding of biochemistry that did not yet exist. Santorio was trying to figure out metabolism.
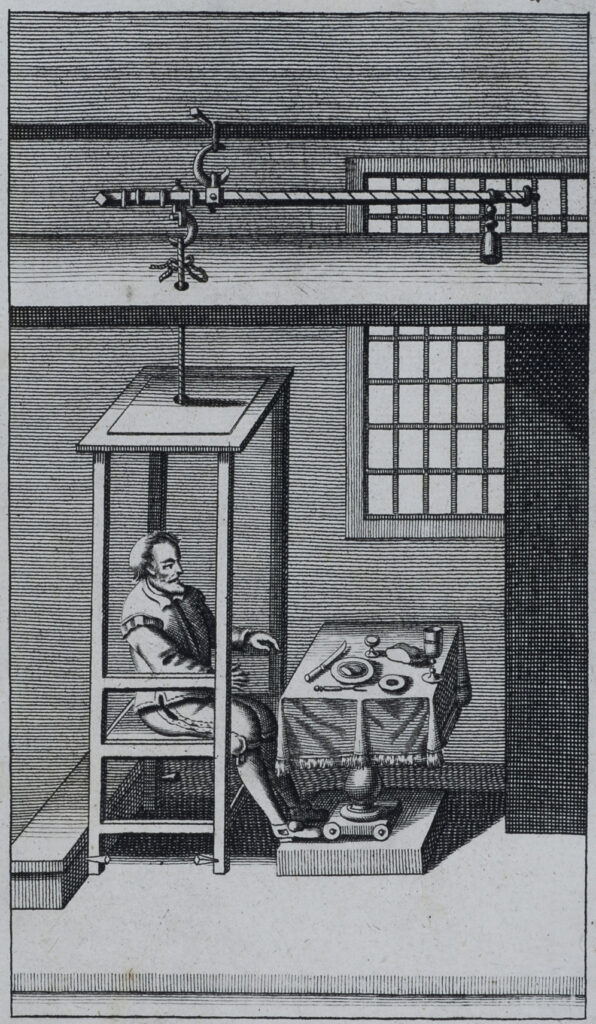
Illustration from a 1723 English edition of Italian physician Santorio Santorio’s Medicina statica, showing the apparatus he built to weigh himself and what he consumed and excreted.
Most of us think of our metabolism purely in terms of how much dessert we can eat before gaining weight. But the word actually refers to something much more complex and profound—namely, the life-sustaining chemical processes that occur in a living organism’s cells. The term itself was coined in 1839 by German physiologist Theodor Schwann, who also happened to be one of the first people to realize all living things are made up of cells.
We now know there are two main types of metabolism: catabolism, the breaking down of complex molecules (releasing energy in the process), and anabolism (well known to body builders), the building up of molecules. Broadly speaking, you can think of catabolic metabolism as the way to get energy out of food, and the catabolism of food really can be compared to a very slow-burning internal fire. When we eat, digestive enzymes break fat, carbohydrate, and protein down into their building blocks—fatty acids and glycerol, glucose, and amino acids, respectively. Glucose is our primary energy source, but under the right circumstances we can use any of these molecules as energy.
The next step is to use these molecules to synthesize our bodies’ basic form of fuel: a molecule called adenosine triphosphate, or ATP. ATP is to our bodies what dollars are to the American economy: it’s what makes us run, providing the energy for everything we do. We can’t store ATP, however, so when we break it down and use its energy, its by-products are recycled back into new ATP. That’s why, amazingly, average humans turn over their body weight in ATP every single day.
Like fire, ATP production requires oxygen and a fuel source—molecules derived from the food we’ve digested. Each macronutrient—fat, carbohydrate, protein—takes a slightly different pathway in the first stages of cellular respiration, but by the end of the process the results are the same: you end up with ATP plus some by-products, such as carbon dioxide, heat, and water. And that is the long-awaited answer to Santorio’s question: the missing weight is mostly water.
I’m writing about metabolism like it’s obvious: you digest food, perform cellular respiration, and produce ATP, duh. But this process actually wasn’t decoded until the 20th century. Figuring it out took hundreds of years and required innumerable steps, including recognition of the connection between the burning of fuel and the digestion of food.
One of the most important steps in the process occurred in the late 1700s when a French tax collector and chemist named Antoine Laurent Lavoisier conducted a groundbreaking experiment to explore the question of why we breathe. At the time, many people believed that the point of respiration was to cool the heart and blood. But Lavoisier wondered if breathing might instead be part of some sort of combustion occurring within the body.
To investigate this idea he worked with the astronomer Pierre-Simon Laplace to build the world’s first ice calorimeter, a device that could measure the amount of heat produced when something was burned. It worked like this: first, they stuck a guinea pig into the ice chamber and measured the amount of oxygen the rodent consumed and the heat and carbon dioxide it produced over a certain period. Then they burned a piece of carbon in the calorimeter that they knew would produce the same amount of carbon dioxide as the guinea pig. Their goal was to see if the heat produced by the combustion of the coal and the respiration of the guinea pig were the same.
I never discovered the fate of those poor, frostbitten guinea pigs, but the experiment resulted in a scientific breakthrough: the ratio of heat to carbon dioxide for the guinea pig and its equivalent hunk of carbon was essentially the same. This, Lavoisier correctly concluded, meant that respiration is part of a very slow internal combustion process; there was some connection, in other words, between breathing and fire.
Most of us think of our metabolism purely in terms of how much dessert we can eat before gaining weight. But the word actually refers to something much more complex and profound.
Lavoisier took his experiments a step further. He outfitted his assistant, Armand Séguin, with a sealed suit made of rubber-coated taffeta designed to prevent air or humidity from escaping. Lavoisier then glued a tube to Séguin’s lips to deliver pure oxygen to breathe. His goal? Find the correlation between the oxygen Séguin inhaled and the carbon dioxide and water (measured by the liquid trapped in the rubber suit) he produced—in other words, what we’d now refer to as his metabolic rate. In November 1790 Séguin and Lavoisier concluded that “respiration is only a slow combustion . . . similar in every way to that which takes place in a lamp or lighted candle, and, from this viewpoint, breathing animals are actual combustible bodies that are burning and wasting away.”
Who knows what else Lavoisier might have discovered, but unfortunately his life took a tragic turn. His work as a tax collector caught the attention of the leaders of France’s postrevolutionary Reign of Terror; on Thursday, May 8, 1794, hours after his trial, he was led to Paris’s Place de la Révolution and beheaded. “It took but an instant to chop off his head,” lamented the mathematician Joseph-Louis Lagrange, “yet a hundred years will not suffice to produce one like it.”
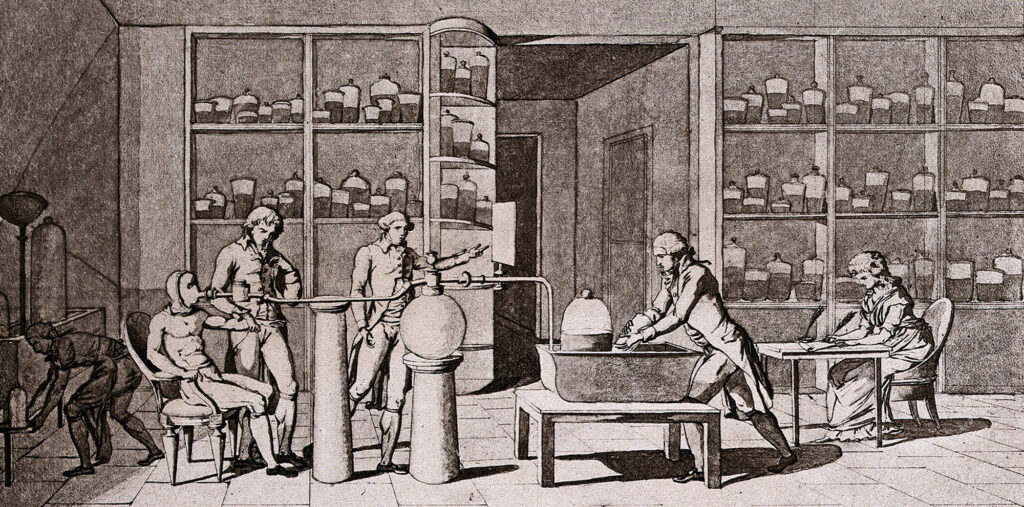
An illustration by Marie-Anne Lavoisier of her husband’s experiments in respiration. Lavoisier’s experiments led him to conclude that respiration was related to combustion.
Nonetheless, over the next century the basics of modern nutritional science began to settle into place. In 1827 Prout—the same guy who realized that gastric juice contains hydrochloric acid—proposed that food could be broken down into three “staminal principles,” which we now know as macronutrients: carbohydrate, protein, and fat. Later in the same century the great German chemist Justus von Liebig proclaimed he had figured out staminal principles: fat and carbohydrate were energy sources, and protein was used to repair or replace damaged tissues. Liebig believed it didn’t matter what foods the protein, carbohydrates, or fat came from. Throw in some salt and water, and presumably you had everything you needed to thrive. (No one knew what the minerals in food were for, and vitamins and other micronutrients weren’t even suspected.)
In the late 1880s a cast of famed German nutritional scientists that included Max von Pettenkofer, Carl von Voit, and Max Rubner took Lavoisier’s and Liebig’s research a step further. They did so by building the world’s first human-sized respiration apparatus, an 8-foot-square iron cube that looked like a boiler and was basically a more sophisticated version of Lavoisier’s rubber suit. With this device they put male test subjects through different circumstances and activities, analyzing their needs for both oxygen and food. Their goal was to answer questions that still baffle us today: What should we eat? And how much of it?
Not so long ago I found myself trying to answer that second question as I sat at my dining-room table with a plastic mask strapped to my face and a stranger named Harvey listening to me breathe. A tube led from my mouth to a small machine placed between us on the tablecloth that generated a real-time graph on Harvey’s computer screen; the room was silent except for my exhalations and the ticking of a clock.
Harvey was a sales representative for the MedGem calorimeter, a modern version of the respiration apparatus developed by those 19th-century German scientists. The MedGem measures your metabolism by analyzing your exhalations; since oxygen is metabolized into carbon dioxide, the more CO2 you’re exhaling, the more calories you’ve burned. Harvey was helping me determine my resting metabolic rate—that is, the number of calories my body requires to stay alive without any exercise or movement.
Harvey had my result after just 10 minutes of awkward silence: my resting metabolic rate appeared to be approximately 1,400 calories.
This happens to be fewer calories than are present in a 10-pack of White Castle mozzarella sticks. I find this fact itself distressing, but I’m simultaneously amazed at its specificity, especially considering what the term calorie actually means: derived from the Latin word calor (heat), it’s a unit of measure for stored heat, originally used to describe the fuel efficiency of steam engines. (Technically speaking, one food calorie—also known as a kilocalorie—is the amount of energy required to raise the temperature of a kilogram of water by 1°C.) For other scientists calories have become obsolete, having been replaced by other units of energy, such as joules. But in the world of nutritional science and public education, the calorie remains king.
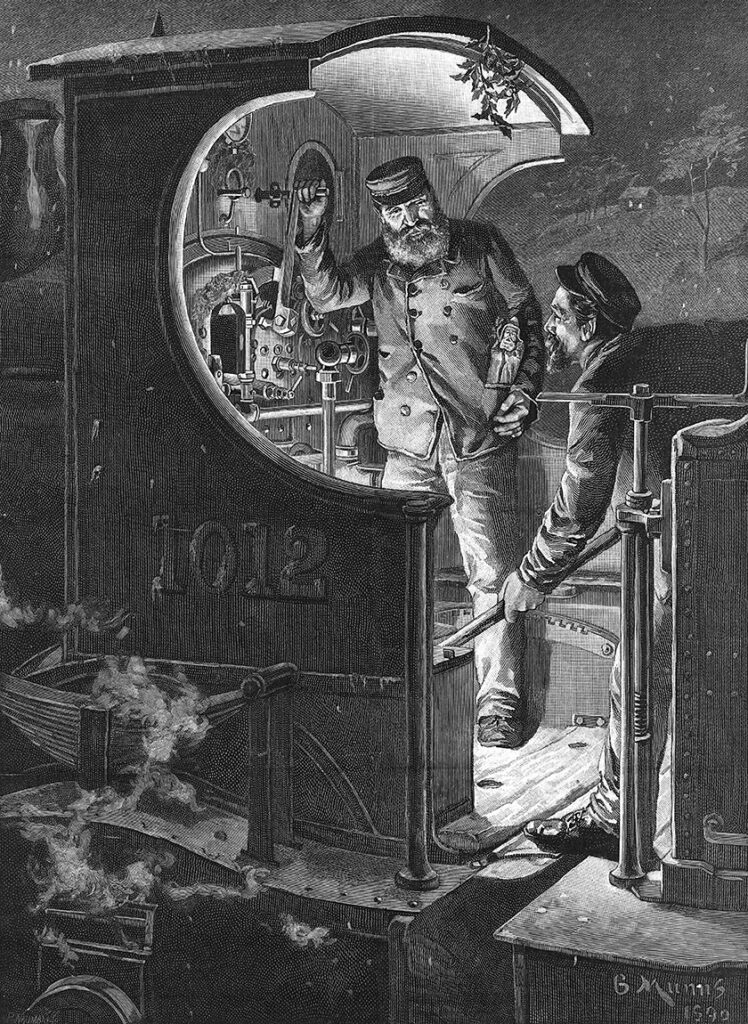
A stoker shovels coal into a steam train’s firebox in this engraving from the Illustrated London News from 1891. Calories were first conceived as a way of measuring the efficiency of steam engines. While other units eventually replaced calories as a measure of energy, in nutritional science they stuck.
The calorie’s staying power is in part due to the work of an American named Wilbur Olin Atwater, who was a pupil of those German researchers and one of the United States’ most influential nutritional researchers. Atwater was born in 1844 in Johnsburg, New York, and originally intended to be a civil engineer. Instead, he earned a PhD from Yale University in agricultural chemistry and spent two years studying agricultural and physiological chemistry in Germany. Eventually, Atwater switched his focus to analyzing the chemistry of food and traveled to Munich where he learned the new German techniques for analyzing the macronutrient contents of food, knowledge he immediately put to use on his return home.
Back in the United States, Atwater busied himself evaluating the calories and macronutrients in hundreds of common foods and updated the German nutritionists’ calculations for how many calories per gram each macronutrient contains. (We still use Atwater’s measurements today: protein and carbohydrates have about four calories per gram, and fat has about nine.)
Eventually he finagled funding to build the country’s first human respiration apparatus at Wesleyan University in the mid-1890s. He wanted to figure out “how much of each of the different nutrient ingredients of food . . . a man actually consume[s] in his body under different conditions of rest and work.”
Atwater’s respiration calorimeter was a little different from the MedGem that measured my metabolism in 10 minutes. Here’s how he described it in the Century Magazine in 1897:
Most of the experiments done in German respiratory calorimeters had lasted for fewer than 24 hours, which already sounds like a long time to endure what the editors of the Century Magazine called “harmless vivisection.” But Atwater’s experiments were much longer, topping out at a mind- and potentially body-numbing 12 days.
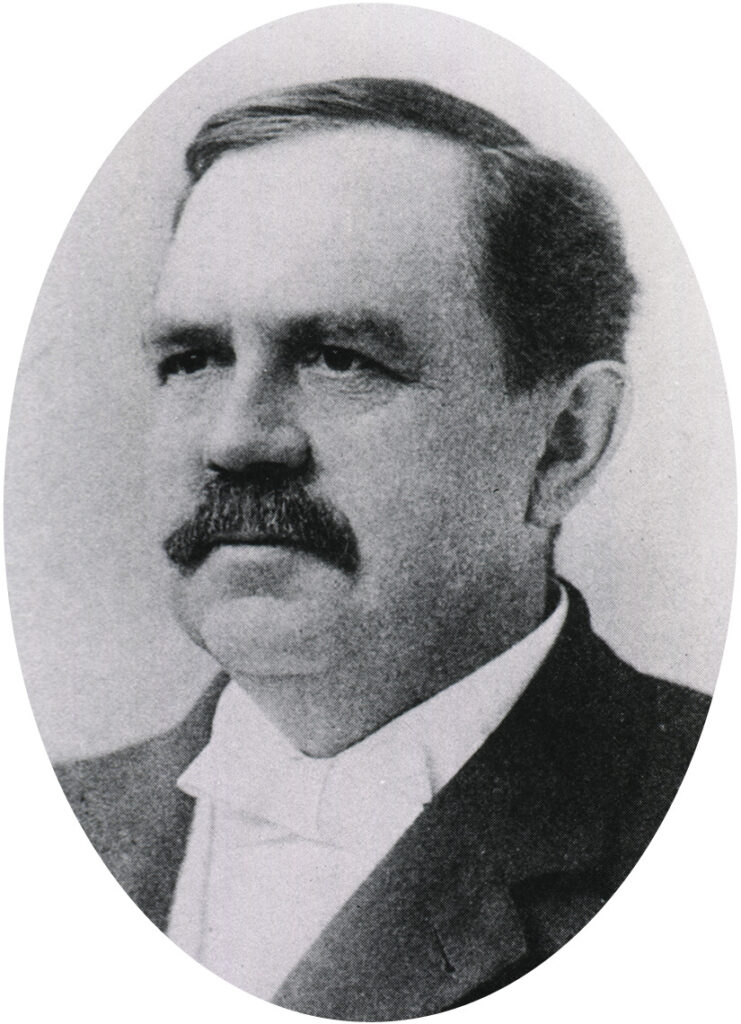
Undated photograph of American chemist Wilbur Olin Atwater, whose research into the calorie content of different foods laid the groundwork for our calorie-counting culture.
In these first experiments Atwater used the calorimeter to measure how much energy a man consumed in different activities. In addition to a day and a half each of warm-up and cool-down, the aforementioned 12-day experiment included 3 days of near absolute rest (“I tried to vegetate, and think I succeeded pretty well,” wrote the participant), 3 days “engaged for eight hours a day in raising and lowering a heavy weight,” and 3 days participating in “severe mental labor,” consisting “partly in computing the results of previous experiments and partly in studying a German treatise on physics.”
Through the use of his calorimeter Atwater was able to examine human metabolism and calorie expenditure more thoroughly than had ever before been possible. The next time you read a story about how exercise raises your metabolic rate or how many calories you burn while chewing on a leaf of lettuce, think back to Atwater and his giant copper box: this is how they figured it out.
But that was far from Atwater’s only contribution to our modern attitude toward food. At the time of Atwater’s research no one knew that calories derived from animal fat, for example, might affect the body differently from the calories in chocolate chip cookies. Food just had the two purposes described by Liebig: to provide energy and, through protein, to repair and rebuild tissue. So Atwater and his German colleagues reached what seemed like a rational conclusion: if food’s sole purpose was to provide protein and calories, and if calories were interchangeable, then the best diet would be one that was the cheapest and most protein and calorie dense.
ATP is to our bodies what dollars are to the American economy: it’s what makes us run, providing the energy for everything we do.
In the mid-1880s, before he had a calorimeter of his own, Atwater began writing articles for a lay audience in hopes of helping the public choose a better diet. In 1887 and 1888 he published a series of articles in the Century Magazine called “The Chemistry of Foods and Nutrition” that still influences us; in it, he encouraged people to think of food not simply as nourishment but as a sum of its parts. This idea represented a sea change in how Americans viewed food, which Atwater summarized perfectly in a later article from 1892:
He was proposing the very attitude we have toward food today.
Atwater’s attempts to educate the American public turned out to be well timed. Thanks to the economic depression of the 1870s and the rise of industrialization, the United States in the 1880s was facing huge social, economic, and political problems. Rapidly expanding slums, violent strikes, and the threat of an uprising by impoverished workers had brought national attention to the question of how to improve the living conditions of the poor (if for no other reason than to prevent them from revolting). Some reformists argued for wage increases; others hoped to help the lower classes while still adhering to the principles of economic laissez-faire.
Atwater’s own message had economic undertones, but these soon became much more explicit thanks to a Boston businessman named Edward Atkinson, who read Atwater’s series of articles and was struck by the idea that Americans, by purchasing expensive cuts of meat or low-calorie, low-protein foods such as vegetables, were wasting money on food that was not nutritionally necessary. The two began to collaborate and, with a chemist named Ellen Swallow Richards, they decided that an economically friendly solution to the social problem was staring them in the face: to improve the living conditions of the poor, you needed to increase their disposable income, and to do this you encouraged them to eat cheaper food.
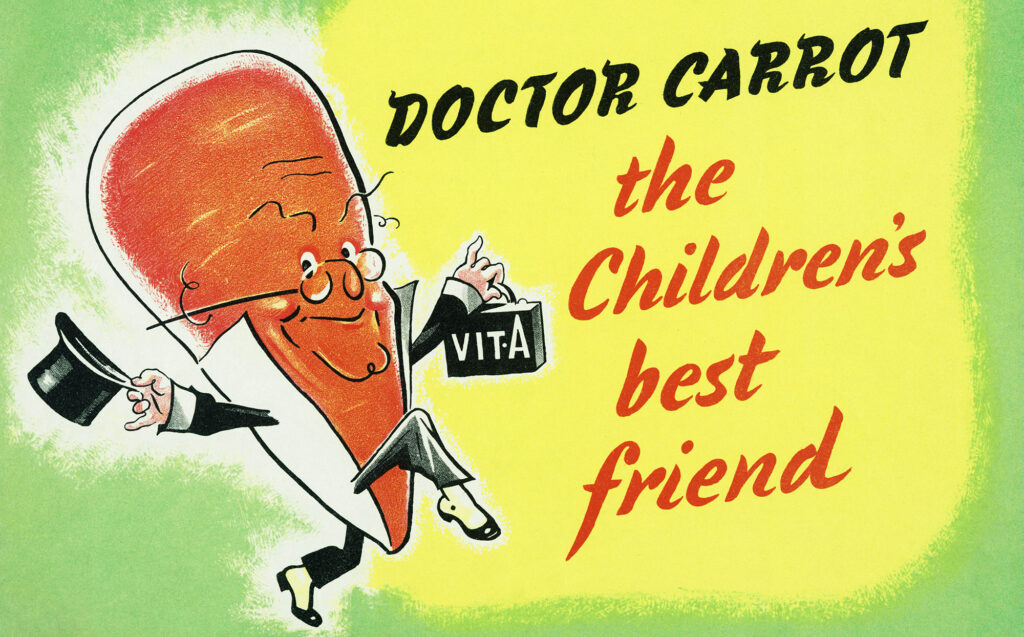
A stoker shovels coal into a steam train’s firebox in this engraving from the Illustrated London News from 1891. Calories were first conceived as a way of measuring the efficiency of steam engines. While other units eventually replaced calories as a measure of energy, in nutritional science they stuck.
Atwater and his colleagues argued that since (in their belief) calories were basically interchangeable as sources of energy, then the poor should be eating the cheapest calories possible; every penny saved on food was a penny they could spend somewhere else. “Half of the earnings of wage workers in this country and in Europe is spent for food,” Atwater wrote in 1895. “The health and strength of all are intimately dependent upon their diet. Yet most people understand very little about what their food contains, how it nourishes them, whether they are economical or wasteful in buying and preparing it for use, and whether or not the food they eat is rightly fitted to the demands of their bodies.”
To prove this point Atwater published tables that compared the calories in various foods; one pound of turnips, he pointed out, contained only 139 calories compared with the 3,452 calories provided by the same amount of very fat pork. To Atwater and his followers an apple was clearly inferior to a Jimmy Dean’s pancake-wrapped sausage stick.
We now know that if you followed Atwater’s produce-hating advice too closely, you’d put yourself at risk for scurvy, beriberi, pellagra, and other micronutrient-deficiency diseases. So it’s good Atwater and his colleagues’ recommendations never really caught on. As the biochemist Elmer McCollum, considered the discoverer of vitamins A and B, later concluded, “From the point of view of the peace of Atwater’s soul, it was a very lucky thing that he never had to come face to face with men or animals whose nourishment was set up according to his directives. It must also be considered a piece of very good luck indeed that the housewives of America made no attempt to feed their families according to his advice.”
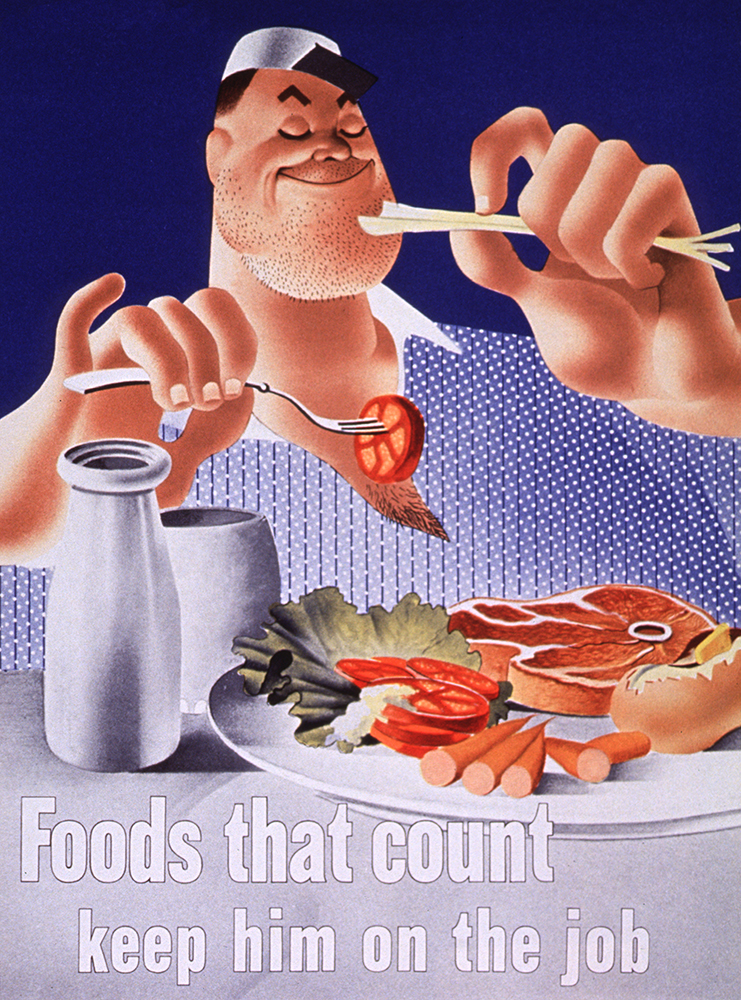
Even though Atwater’s nutritional advice was never followed en masse, his work caused a revolution in the way the public thought about food. His articles were the first time many people had heard of such things as carbohydrates, proteins, and fat. Reading the articles now is like visiting an entirely different world.
Yet Atwater’s articles also seem strangely familiar; the philosophy he proposes is one that—for better or for worse—we’ve wholeheartedly embraced. We count calories. We buy foods based on the nutrients they contain. We debate ways to create social change through diet. We emphasize “health”—and chemistry—over taste. Though we’re not consciously aware of it, Atwater comes along with us every time we visit the grocery store.
It’s tempting to think we’ve resolved the question of how the food we eat affects our health. But in reality many questions remain unanswered—including whether calories are in fact interchangeable—and many of our most cherished beliefs may turn out to be untrue. As is always the case with science, we should constantly challenge “common knowledge.” And as these historical examples make clear, it would be unwise for us ever to conclude, in nutrition or anything else, that we’ve got everything figured out.