Cecelia Bavolek had a hole in her heart the size of a half-dollar.
The hole had been there since birth, and her doctor warned her family it would someday require surgery. Now, in the fall of 1952, as she began her freshman year at Wilkes College, she was finally feeling its effects. Her heart was enlarged and would sometimes beat irregularly. Her breath labored as she walked up a flight of stairs. By January she was hospitalized with pneumonia. Blood streaked her cough.
After a second hospital stay in March, Bavolek’s doctors delivered their verdict. As blood rushed into the upper chambers of her heart, half was leaking from her left atrium to her right, cycling back to her lungs rather than other parts of her body. The resulting imbalance left her weakened and lightheaded. She had made it to 18 without symptoms, but if the hole wasn’t repaired, she might not make it to 19.
Operating on the heart, however, would be like repairing a car’s engine while screaming down the highway at full speed. Doctors gave Bavolek two options to attempt the high-stakes maneuver.
The more reliable method involved cloaking her in refrigerated blankets, which would slow her heart’s beat to a crawl and stifle her body’s demand for oxygen. Doctors would take her right to the edge of a frigid death, but if all went to plan it would give them time to mend the gap.
The other option sounded even more far-fetched, like something out of a science-fiction tale: Doctors would shut down her heart and lungs completely and use a machine to carry on their functions outside of her body.
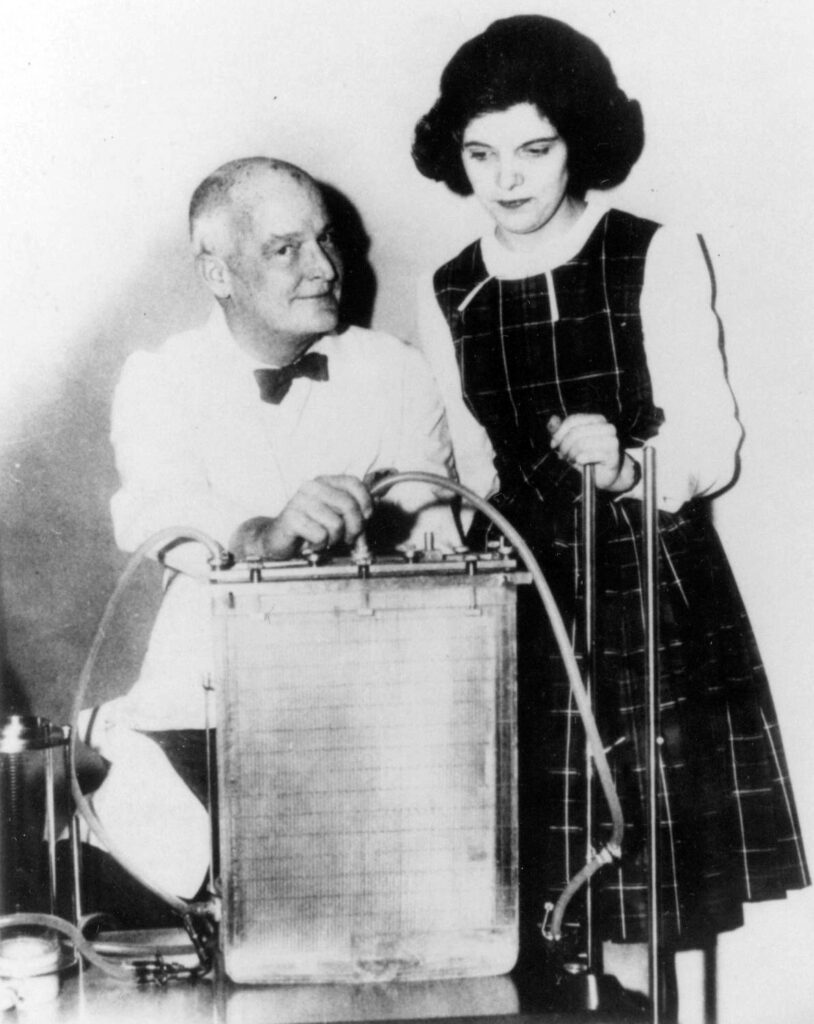
Bavolek’s family doctor referred her to the Philadelphia surgeon behind the futuristic device. John “Jack” Gibbon was known as a man intent on exploring the horizon of medical possibility. When Gibbon presented his idea at a 1939 meeting of the American Association for Thoracic Surgery, surgeon Leo Eloesser, straddling the line between awe and skepticism, compared it to “the fantastic tales of Jules Verne, who anticipated impossible accomplishments which perhaps one day will become reality.”
Gibbon had spent the years since then working toward that reality, which he called the extracorporeal circuit. It was a device that could stand in for the heart and lungs, bringing that racing engine to a halt to make its repair possible.
With the help of engineers at IBM, he had produced a million-dollar machine whose tangle of mesh screens, pumps, and rollers had proven effective when operating on dogs and cats. But no human had yet survived surgery with their blood coursing through the device. A year earlier, the first patient connected to it had died.
Bavolek, though, couldn’t stand the idea of being put on ice like some fish at the grocery store, so she threw her lot in with Gibbon, following what she later called a “youthful hunch.”
“It must have taken great courage on Cecelia’s part to give her consent to the procedure,” Gibbon admitted.
Gibbon’s persistence and Bavolek’s bravery had brought the field of surgery to its final frontier, where centuries of medical knowledge and technological advances offered a fix for the unfixable. He had been doubted for years, and she would be treated as an oddity if it worked: the woman whose heart was stopped by doctors and lived to tell the tale. Together, they prepared to push the boundaries of surgery, dragging its long past into a future few had imagined.
Perhaps not understanding exactly how the machine worked, Bavolek’s physician offered an ironic bit of advice before she went under: “Celie, just keep on breathing and you won’t die.”
Instead, she handed that responsibility over to a stainless-steel contraption the size of an upright piano, and Gibbon got to work.
Gibbon’s Eureka Moment
Gibbon’s journey to that Philadelphia operating room had begun 23 years earlier in Boston. In October 1930, he was a 27-year-old surgical research fellow at Harvard, working at Massachusetts General Hospital. A patient on his floor was 15 days out from a gallbladder operation when she developed a massive pulmonary embolism. There was little doctors could do to help her.
Surgery to remove the blood clot from her lungs would be a last-ditch effort—it had not yet been performed successfully in the United States. Edward Churchill, the doctor overseeing Gibbon’s work, said the procedure was more like a postmortem examination than an operation; without the lungs feeding it oxygen, there was little chance her heart would keep pumping through the surgery.
All through the night Gibbon sat by the patient’s bedside, dutifully recording her blood pressure and pulse every 15 minutes, hoping to avert the operation. Once she fell unconscious at 8 a.m., it took just over six minutes for Churchill to perform the embolectomy. But she couldn’t be revived.
The memory of that vigil haunted Gibbon—watching the woman march toward death, her blood growing darker and her veins more distended as he longed for a way to remove the blue blood from her body, reoxygenate it, and return it to her, red as a rose.
But there were happier days in Boston, too. He met his partner in marriage and science in Churchill’s lab. Mary Hopkinson, known to her family and friends as Maly, was born in nearby Manchester, Massachusetts. Her father, Charles Hopkinson, was a renowned portraitist. Her family was well-to-do, but she didn’t come from a long line of doctors like Jack. Instead, she had developed her interest in medicine during a winter in Paris with her cousin, Francis, and Francis’s doctor husband. Each night Maly would unspool new questions about medical research. As her host gamely answered, a new world opened up to her.
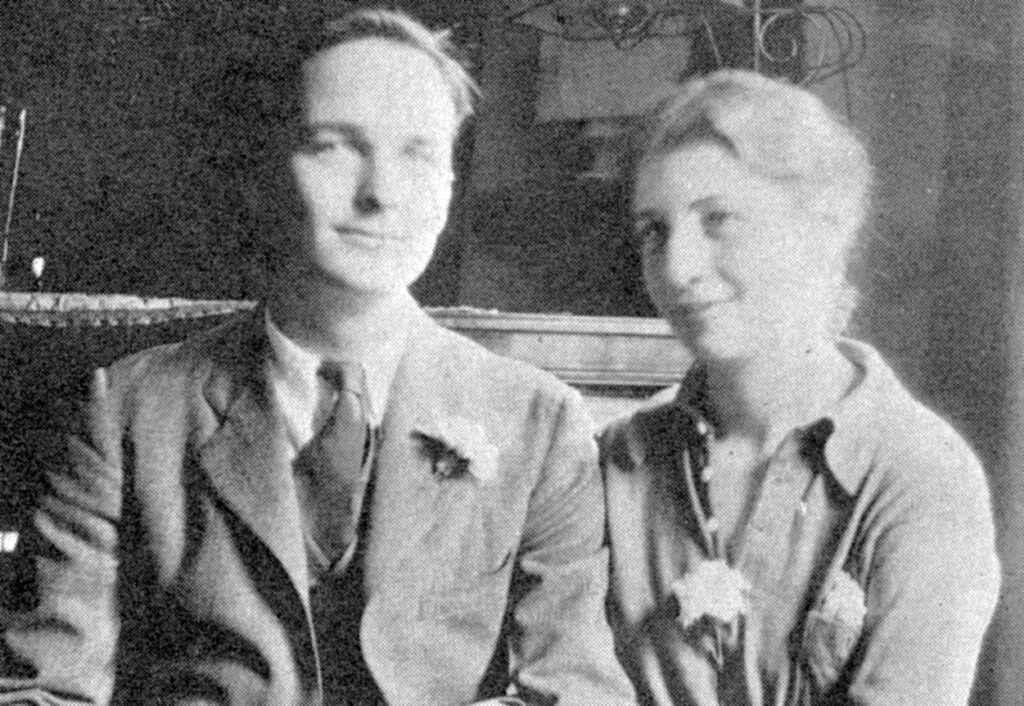
Undaunted by her lack of a college degree or experience, she returned home and applied for a job at Harvard Medical School. Churchill, an unconventional thinker, brought her on as research technician. He was thrilled to train someone who didn’t have a medical education to unteach. By the time Jack arrived three years later, Maly was an expert in laboratory procedures involving animal research. She introduced him to experimental surgery, and they began simulating pulmonary embolism in cats to understand how it could be treated. Maly ensured the consistency of their procedures and data collection.
The pair married in March 1931 and moved to Jack’s hometown of Philadelphia. Maly remained Jack’s assistant at the University of Pennsylvania, and the couple started raising a family. Jack practiced thoracic surgery each morning. In the afternoon, the couple pursued a range of research experiments, but none could distract Jack from a nagging idea: If doctors could use a mechanical apparatus to temporarily carry on the functions of the heart and lungs, they would be able to operate on the heart “under direct vision in a bloodless field.” They would be able to breach the final frontier.
Cats, Dogs, and Cardiac Surgery
“The road to the heart is only two or three centimeters in a direct line,” surgeon Harry Sherman declared at the 1902 meeting of the American Medical Association, “but it has taken surgery nearly 2,400 years to travel it.”
Sherman was referring to recent attempts to suture damaged hearts, mostly in people whose chests had been punctured or slashed open. The challenge of repairing an impaired heart had vexed physicians as far back as Hippocrates, who recognized the heart as “an exceedingly strong muscle” and offered the first recorded description of a heart attack. Treatment was inconceivable. “A wound in the heart is mortal,” he wrote.
For Sherman, evidence was mounting that the heart—once believed to be so vulnerable that any surgical attempt would be fatal—could withstand “many manipulations, provided they may be done without stopping its action at once.” In 32 cases, doctors had resected patients’ ribs or opened a flap in the thoracic wall to close and dress a wound, typically with silk suture. Nearly half died on the operating table or soon after, but 13 survived. Maybe Hippocrates wasn’t entirely correct.
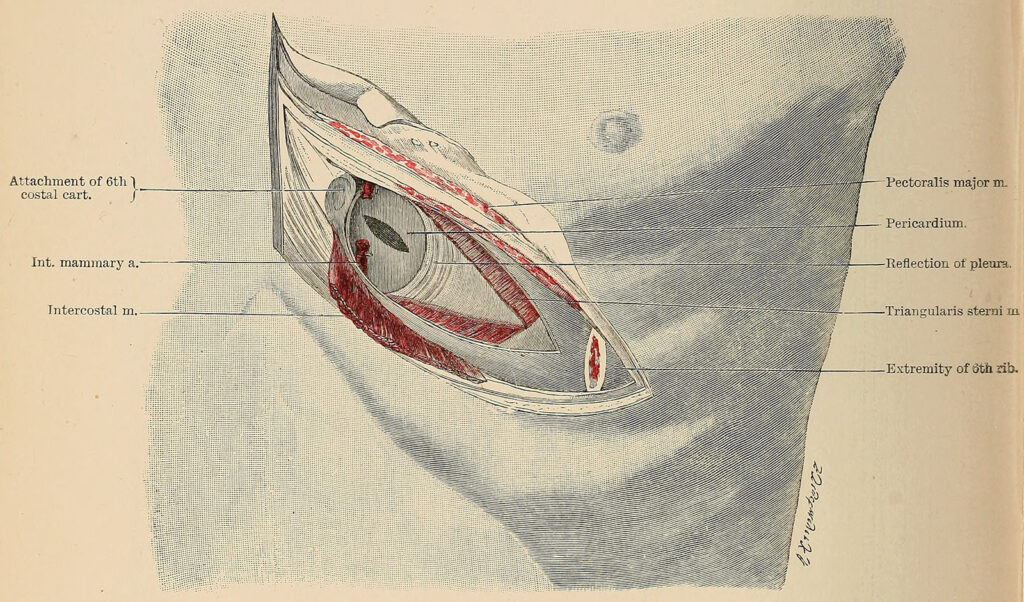
Although Sherman acknowledged that “it is a very unsafe thing to prophesy,” he nonetheless suggested that the next step in the heart’s long history may come soon. Despite his intuition, he could scarcely have imagined where Jack Gibbon would take surgery a half-century further on.
In 1934, Jack petitioned Churchill to take him and Maly back for another year of research. This time, they would focus squarely on building the extracorporeal circuit.
Hardly anyone encouraged the Gibbons in pursuing their dream—not even the nonconformist Churchill—but he accepted their request. The couple returned to Boston with their two young children, Mary and John.
“The general impression,” Maly wrote, “was that it would in all probability be a monstrous waste of the time and energy of a bright young investigator to embark on such an almost impossible task.” A colleague at Mass General advised Jack against the project, urging him to tamp down his ambition if he wanted an academic career in surgery. All but one of Jack’s friends in the medical community discouraged the Gibbons’ dogged pursuit. Nonetheless, the husband–wife team pushed forward.
They started with strays.
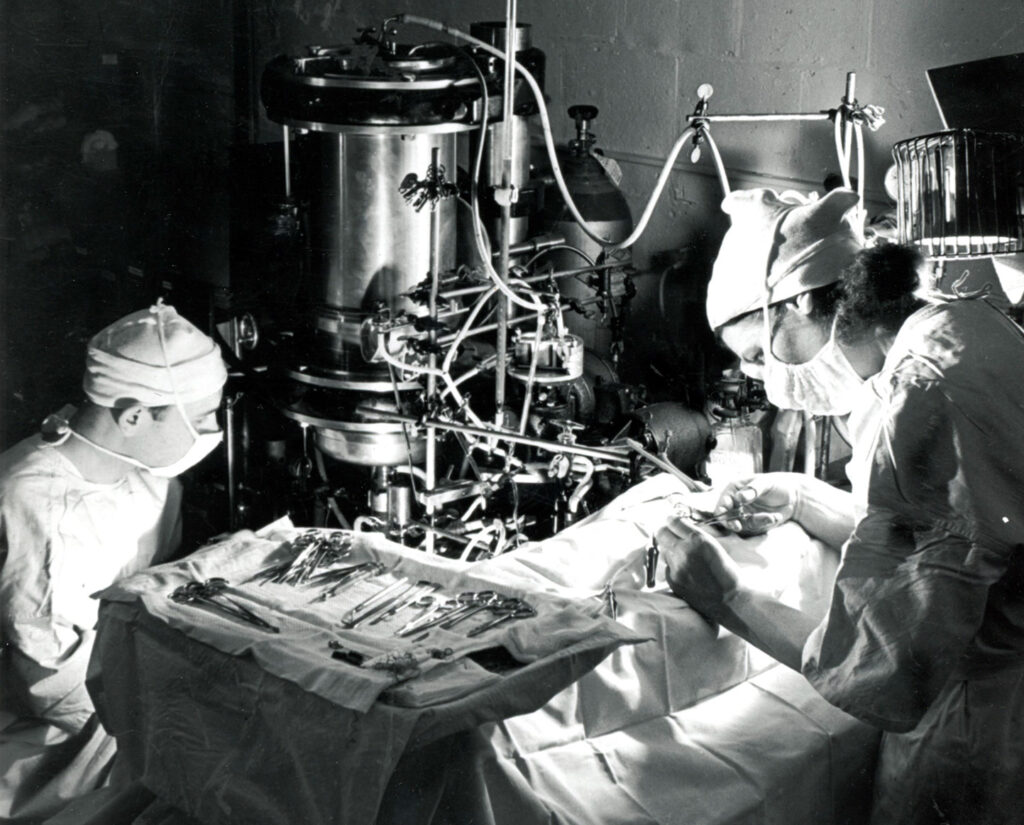
The Gibbons prowled the Beacon Hill section of Boston at night with tuna as bait and a gunny sack to catch the alley cats they found. Smaller animals’ veins were too difficult to access; dogs were too expensive.
To simulate a pulmonary embolism, they clamped a cat’s pulmonary artery, withdrew blood from its jugular vein, and passed the blood through a device where it could pick up oxygen and discharge carbon dioxide before returning to the femoral artery as if it had just traveled through the lungs. The primary challenge was designing an artificial lung that could carry out this complicated gas exchange without incident.
Using a pump with internal valves built from rubber cork and glass tubes, the Gibbons fed the cat’s blood into a rotating vertical cylinder, on whose inner surface the blood created a film. This film gathered oxygen as gravity dragged it toward a glass cup below, which sat in a bath of circulating warm water. A second pump, operating at a cat-friendly rate of 150 beats per minute, pushed the blood back to the cat’s body. Jack may have called it a “Rube Goldberg apparatus,” but it was ferrying the Gibbons toward surgery’s outer limits.
The preparations for these experiments took several hours, and “the things that were apt to go wrong were infinite,” Maly later wrote. If they withdrew blood too quickly, the vein would collapse into the needle. Blood sometimes foamed at the bottom of the cup. Cats given inadequate anesthesia would wake up and sometimes break part of the apparatus.
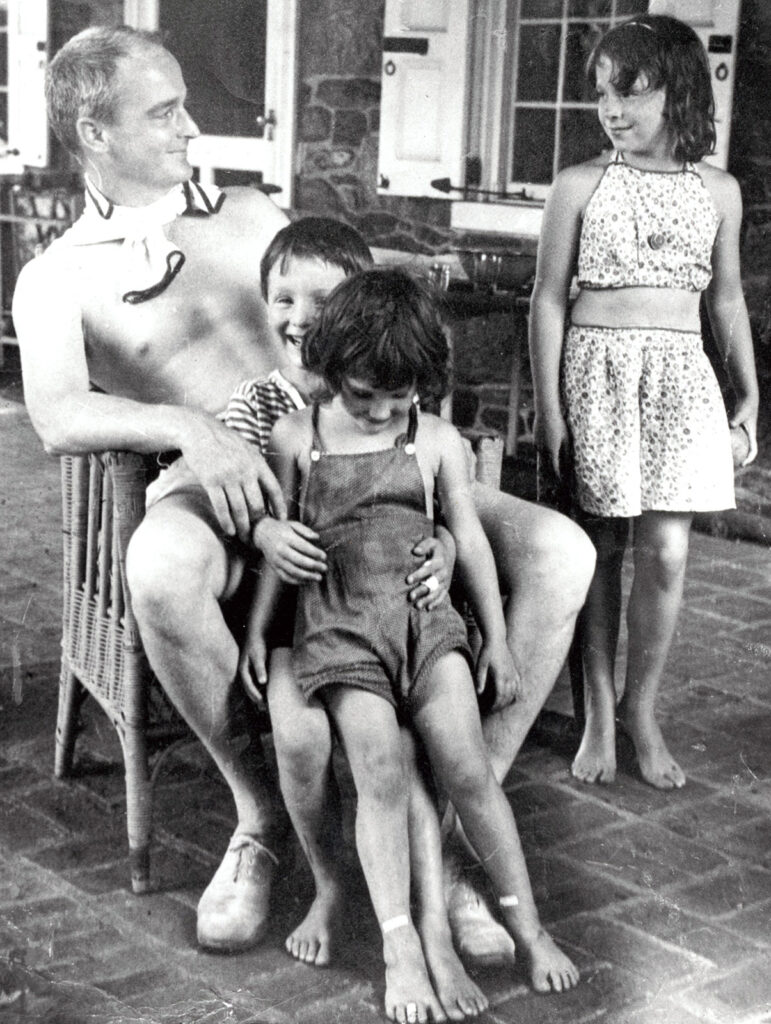
By the end of their year with Churchill, they had gotten these problems under control well enough to keep a cat alive and in a relatively normal physiological state with no blood at all passing through its heart and lungs. The first time they succeeded, the Gibbons danced around the lab, laughing and shouting in celebration.
“Nothing in my life has duplicated the ecstasy and joy of that dance,” Jack later wrote.
The Gibbons returned to Philadelphia and to Penn, where Jack was made a surgical research fellow and Maly his technician. They continued to perfect their device, showing that cats could survive on their machine for as long as 20 minutes. With great understatement, they reported that if they could improve the machine enough to expose a diseased human heart, “the fields of cardiac and thoracic surgery might be broadened.”
But World War II ground the research to a halt when Jack, a reserve officer in the Medical Corps, volunteered for active service shortly after the attack on Pearl Harbor and was sent to the South Pacific. For four years, he left behind Maly and four children—including Alice, born in 1936, and Marjorie, still a toddler. Perhaps most surprising, given his dogged pursuit, he left behind the heart-lung machine.
A Series of Disasters
While Jack was away, Maly kept up her research and kept the family together. She worked with a group of scientists at Penn seeking a treatment for phosgene poisoning.
When he came home from the Medical Corps after the war, Jack put everything aside to practice medicine and focus on his machine. In early 1946, he became professor of surgery and director of surgical research at Jefferson Medical College, and immediately returned to his apparatus, which he now began testing on dogs.
By this time, doctors were performing relatively modest cardiac surgeries with regularity. Their efforts were limited to repairs of openings between arteries or constrictions in the aorta—procedures that didn’t require the heart to be fully exposed and its work outsourced to a machine.
In labs around the country, surgeons sensed a new era was opening and joined a friendly race. While the Gibbons worked to perfect their heart-lung machine, other scientists experimented with hypothermia or the Gross well technique. In the latter, an open-bottomed rubber well was connected to the atrial wall and allowed to fill with a pool of blood, through which surgeons could place sutures.
In 1952, F. John Lewis at the University of Minnesota combined hypothermia and the temporary interruption of blood flow to the heart to perform the first successful open-heart surgery under direct vision. But only a heart-lung machine—and the method of cardiopulmonary bypass that it allowed—offered the requisite time to deal with most congenital heart defects.
Creating such a machine required a safe method of anticoagulation of the blood that could later be reversed, a way to pump blood without destroying red blood cells, and a method for gas exchange. A new drug, heparin, could be used to address the first challenge; pumps used in the dairy and food industry could be adapted to solve the second. The artificial oxygenator was the great mountain to climb.
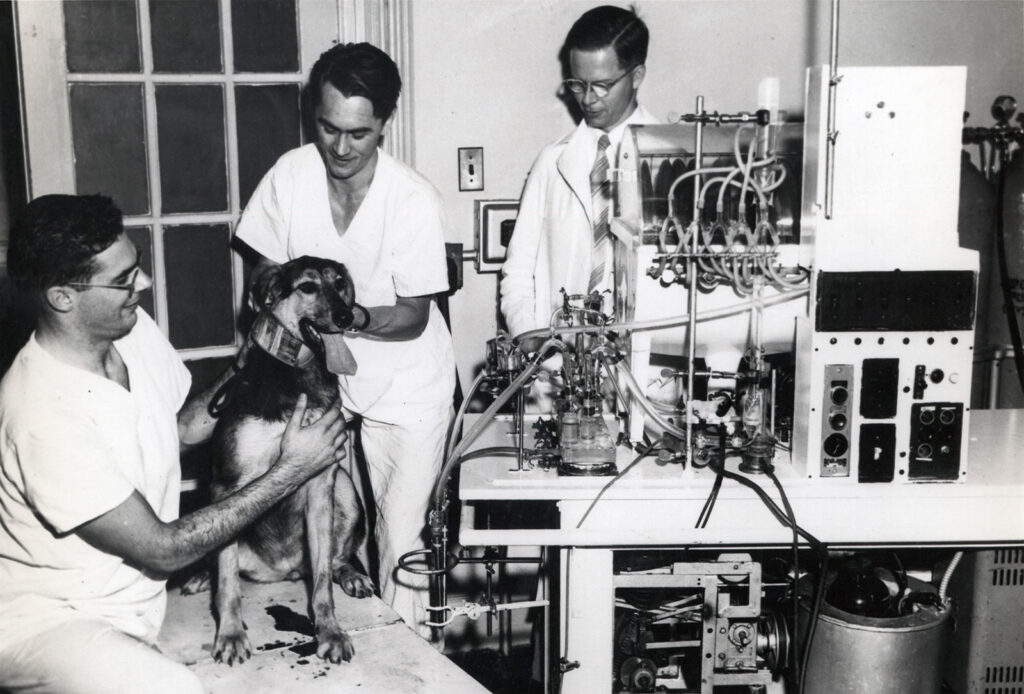
Early attempts to bypass the heart and lungs were “a series of disasters with an appalling mortality rate,” cardiothoracic surgeon William Stoney wrote in a review of the practice’s evolution. Between 1951 and 1955, 18 patients were reportedly operated on using bypass methods; only one survived. Rumors among surgeons suggested there were even more failed attempts that went unreported. Some surgeons began to doubt that the heart could tolerate any type of surgery at all. Others pushed on.
Jack Gibbon’s counterparts—including Clarence Dennis and C. Walton Lillehei at the University of Minnesota and John Kirklin at the Mayo Clinic—each had their own designs, but few had the Gibbons’ extensive laboratory experience to lean on. As they embarked on their trial-and-error processes, the surgeons frequently ran into difficulties with air embolism and postoperative bleeding, among other issues.
Patients paid the price.
William Mustard, at the University of Toronto Medical School, looked for an alternative in rhesus monkeys, using their lungs as an oxygenator. His first seven patients all died in disastrous fashion.
Jack turned instead to Thomas Watson, the chairman of IBM, for help engineering an effective machine. One of Jack’s medical students had a family connection to Watson and offered the two a fortuitous introduction in 1946. Over the next eight years, IBM would pay the entire cost of the three successive machines the Gibbons developed.
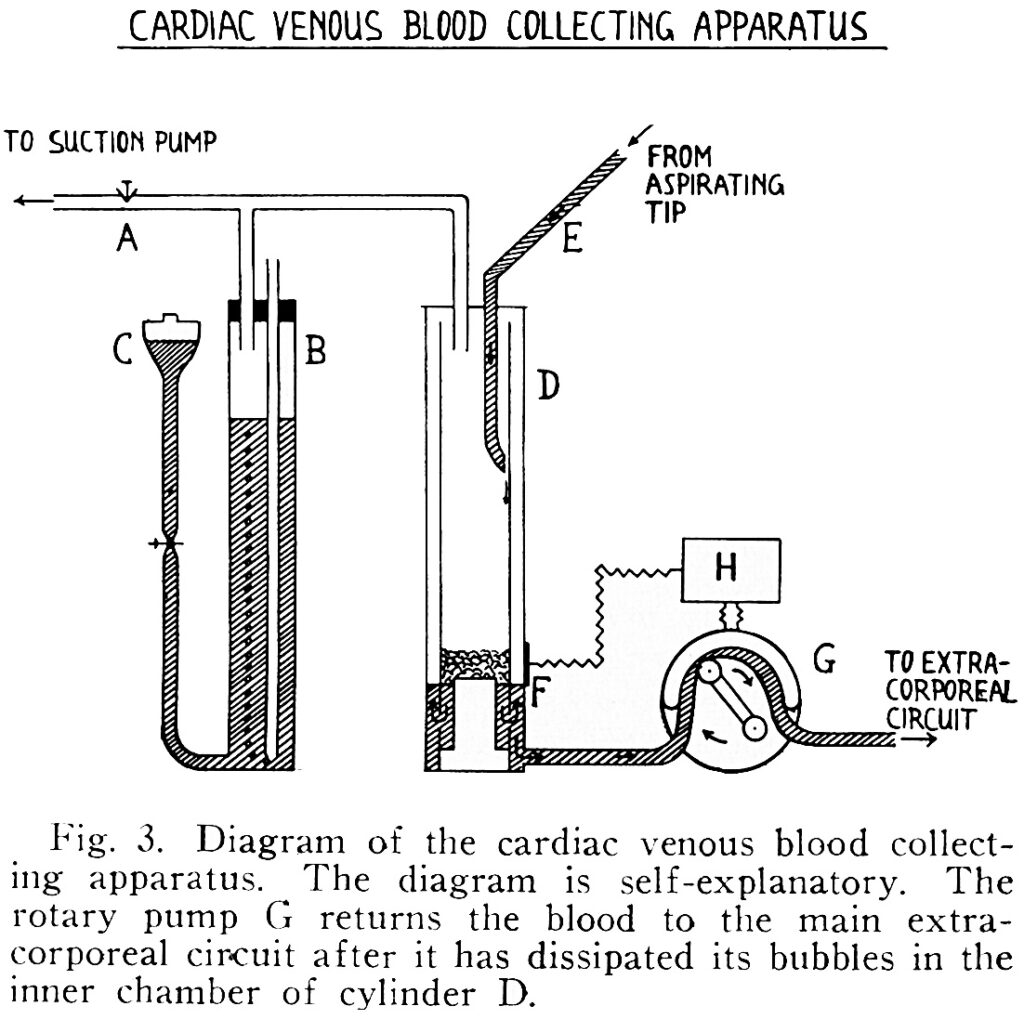
Jack made frequent trips to IBM headquarters as the engineers incorporated his designs into larger models of his machine. What had been done on a shoestring budget with Maly’s help was now being done with only the finest materials in an industrial setting.
IBM engineers helped Jack sort out a number of problems he was having with oxygenation. First, the turbulence created by the revolving cylinder in the Gibbons’ design added too much oxygen to blood, making it foam. To solve this problem, they lined the cylinder with a stainless-steel mesh screen that allowed blood to pass through while achieving just the right level of oxygenation.
But to match the circulation of the average-sized dogs Jack experimented on, the mesh screen would need to be nearly 9 square feet in size. Rather than expand the machine to the size of a room, the team built a series of suspended parallel screens housed within one chamber, expanding its capacity. By 1951 they managed to keep a 20-pound dog alive on the machine for 96 minutes—and perhaps most promising, it survived being taken off bypass.
Jack practiced on dogs for two more years, refining his process and building a second version of the machine before considering an operation on a human.
The Model II, as it was called, was complex, temperamental, and expensive to build. It required multiple technicians to manage it during surgery. It was outfitted with an impressive assortment of safety features, including electronic devices to monitor the volume and pH of blood in the reservoir and a backup, battery-operated generator. The cabinets housing the device used pressurized nitrogen to displace atmospheric gas, reducing the risk that an electrical spark would ignite volatile anesthetic gases.
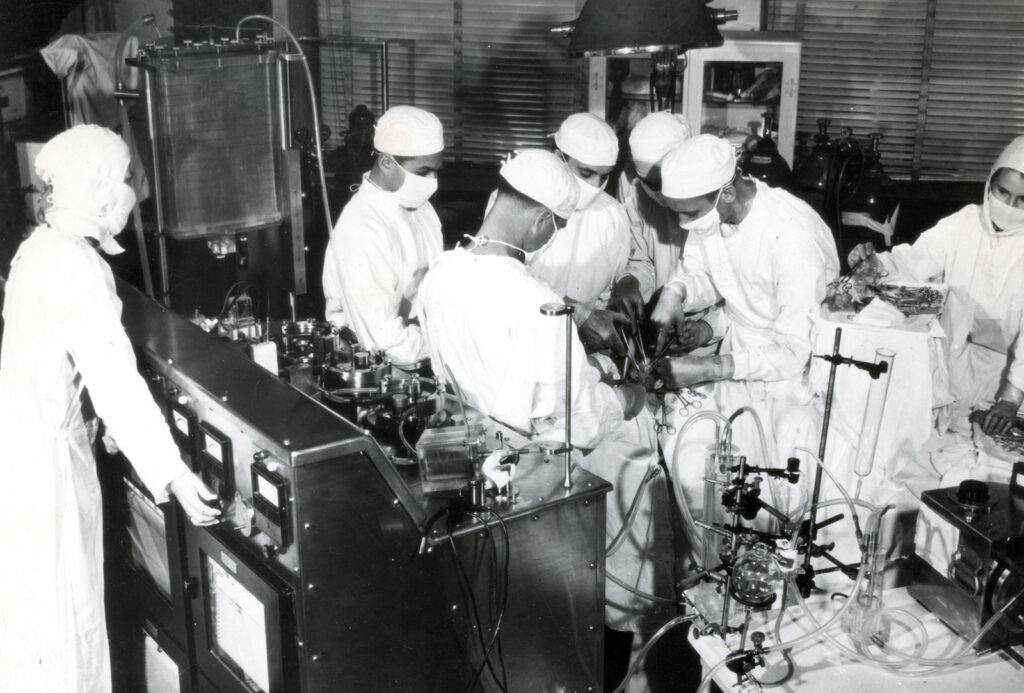
By 1952, two decades after he first had his vision, Jack was confident the device could carry a human through open-heart surgery.
He made his first attempt on a 15-month-old, 11-pound baby, who had been fragile since birth. Doctors believed a hole in the wall separating the upper chambers of her heart was keeping it from working properly. She was so small and her limbs were so swollen with fluid that it wasn’t possible to perform a cardiac catheterization to diagnose her condition with certainty.
At surgery, the machine performed as intended, but as Jack explored her right atrium, he couldn’t find the defect he had expected. She died on the operating table. The issue, discovered during autopsy, was a hole in her aorta. The spectacular machine had done its job, but an inaccurate diagnosis stood in its way. Troubled by the result, Jack sent a resident to the University of Alabama to study cardiac catheterization.
It was the first in a series of deaths that weighed heavily on Jack and would underscore the gravity of Bavolek’s decision.
May 6, 1953
At noon on May 6, Cecelia Bavolek put herself in Jack Gibbon’s hands. A team of doctors, anesthetists, and technicians stepped into the operating room, ready to breach this new horizon.
The doctors placed a needle in her arm to measure blood pressure and inserted intravenous lines into her ankles. After giving her 2 grams of sodium pentothal, Jack opened her chest, slicing through her ribs to expose the heart and its labyrinth of great vessels. Maly watched, taking notes as her husband placed the plastic tubing that would connect Cecelia to the machine.
The Model II’s safety features set it apart from competing devices, Stoney wrote, but they couldn’t account for human error. The oxygenator had to be primed with several units of anticoagulated blood to maintain a film on the mesh screens. But on the morning of Bavolek’s surgery, technicians at the blood bank made a critical mistake—they used only 40% of the heparin required. The error set off a series of calamities.
At 12:55 p.m., doctors began redirecting Bavolek’s blood into the machine, and five minutes later they fully cinched her vena cavae, establishing complete bypass. Within minutes, blood began to foam and leak from the top of the oxygenator. One of the machine’s operators climbed on a stool to hold down the lid and prevent it from overflowing like an overfilled blender.
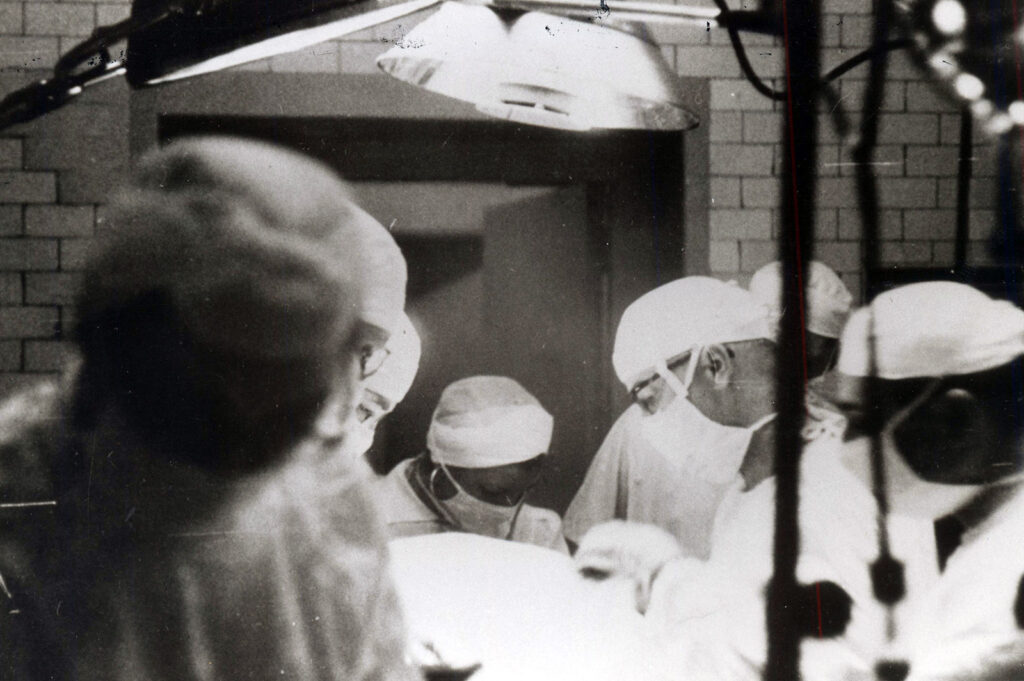
The malfunction caused pressure to build up in the arterial pump tubing, restricting the flow of blood back into Bavolek’s body. Soon, the first three of eight screens in the oxygenator stopped capturing blood. By 1:15, the oxygen saturation in Bavolek’s blood was slightly more than half what it should have been. Fifteen minutes later, it had fallen further still, to 32%. “The blood was black,” one witness said. The blood’s pH was also declining, indicating an alarming buildup of carbon dioxide, threatening respiratory failure.
Jack hurried. He abandoned his plan to use a patch to repair Bavolek’s heart defect and instead closed it with a suture. As her arterial blood pressure sank to dangerously low levels, he shouted to resident Bernard Miller, “Do something!” Miller broke scrub to help resupply the machine with every available donor unit of blood on hand.
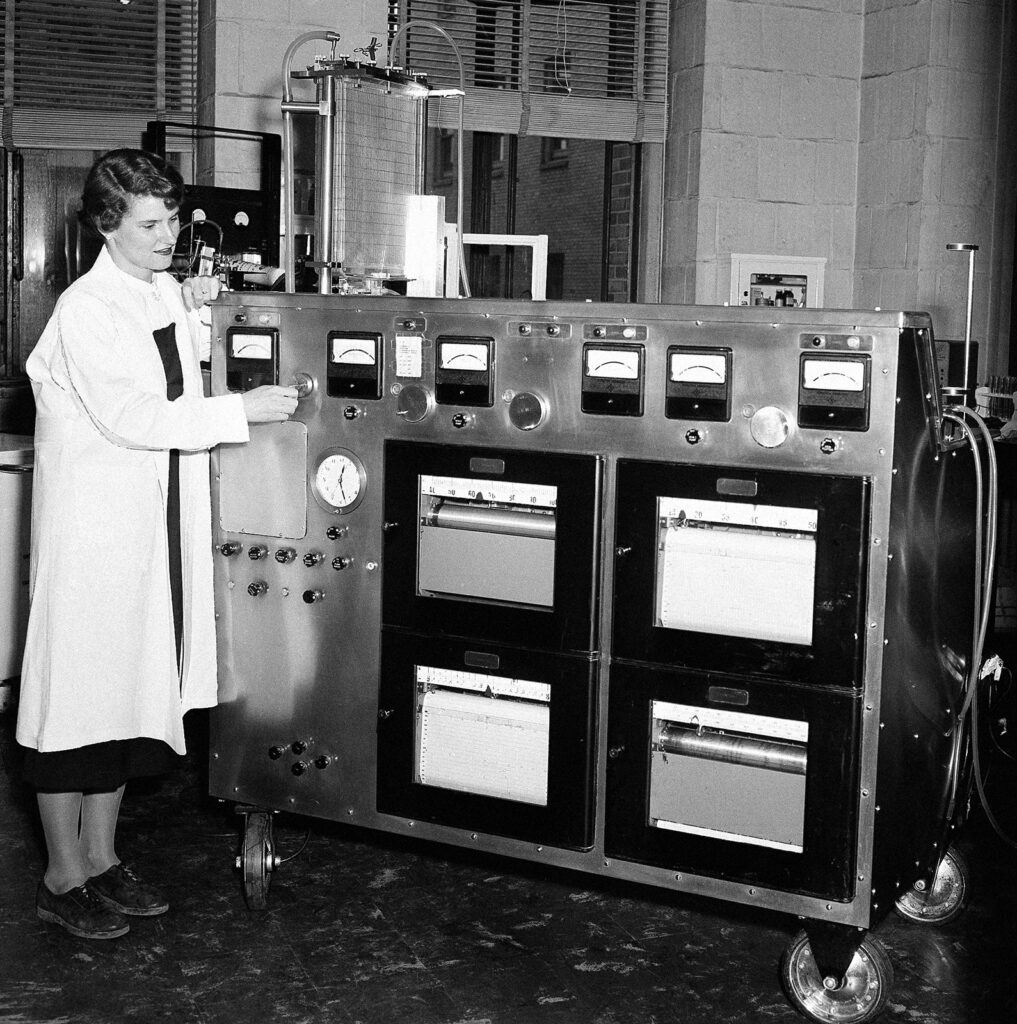
As Bavolek came off bypass, at 1:35, her blood pressure stabilized. Still, her blood had turned acidic and her heart fibrillated, requiring two countershocks. With so much potential harm orbiting her, the doctors stopped anesthesia for the last hour of surgery out of concern for Bavolek’s neurologic health. She awoke on the table while the doctors were suturing her skin—one last cause for panic that harrowing day.
Nine hours after it all began, Bavolek was completely lucid. Thirteen days later, she was discharged in good health.
During a congratulatory phone call from Dennis, his fellow surgeon, Jack remarked, “After we finally got ready, it was ridiculously easy.” But his bravado belied his frayed nerves.
He was so reluctant to relive “the tension and emotional excitement,” he later wrote, that he had his assistants record the operative notes—the only time in his career he passed on that responsibility.
Pandora’s Box
Bavolek’s operation was the only one Jack performed successfully using the heart-lung machine. The surgery was emotionally draining for him, and so, too, were two successive attempts to use the machine.
In the two later surgeries, both on 5-year-old children with heart defects, the machine performed as intended. But still the patients died. One suffered cardiac arrest after her chest was opened and couldn’t survive once separated from the machine. The other had two defects beyond the one Jack had identified; in surgery, he had no bloodless field in which to work. The machine, he now knew, was only part of the fickle work of mending a heart.
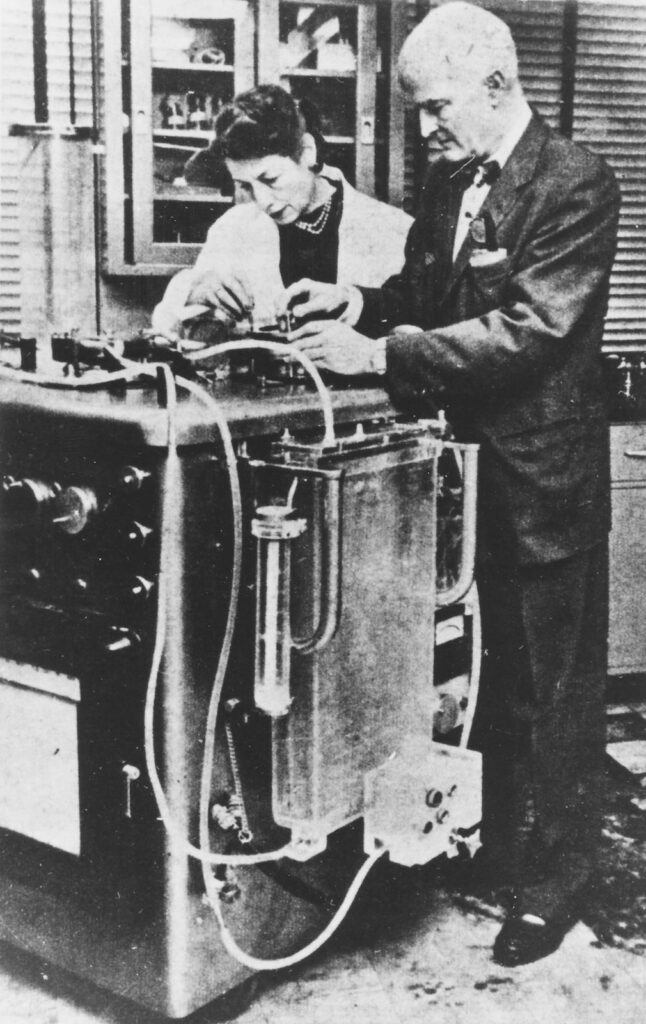
Jack declared a one-year moratorium on the device’s use, but he never returned to it. His focus during all those years in the lab was not on the repair of congenital cardiac lesions; rather, he had wanted to establish that a machine could allow their repair. He had achieved that goal.
The team at IBM delivered a third model in 1954, by which point Jack had entrusted the future of the design to surgeons at the Mayo Clinic, who continued to modify it. The machine remained expensive to build and complex to operate, and other methods of oxygenation, less costly and cumbersome, rose to prominence. It took years of further refinement—and advances in surgeons’ skill and expertise in cardiac procedures—for the heart-lung machine to enter wider use.
But within a decade of Jack’s great leap forward, advertisements for oxygenators could be found in medical journals. His dream was a reality. Seventy years later, more than two million people each year have open-heart surgery around the world.
Bavolek lived a healthy life after the operation, if not a normal one. In its wake, she received incessant inquiries from the media and curious looks from the public. “Oh, you’re the girl they sawed in half,” one woman said to her, as if she was a sideshow act. She grew irritated and exhausted by all the attention. She struggled to find work; employers feared she was barely hanging onto her life. Eventually, she landed a job at Blue Cross and was later named National Heart Queen by the American Heart Association. Ten years after the surgery, she was a secretary and a volunteer at Hahnemann Hospital—mostly with heart patients. She later became a medical technician.
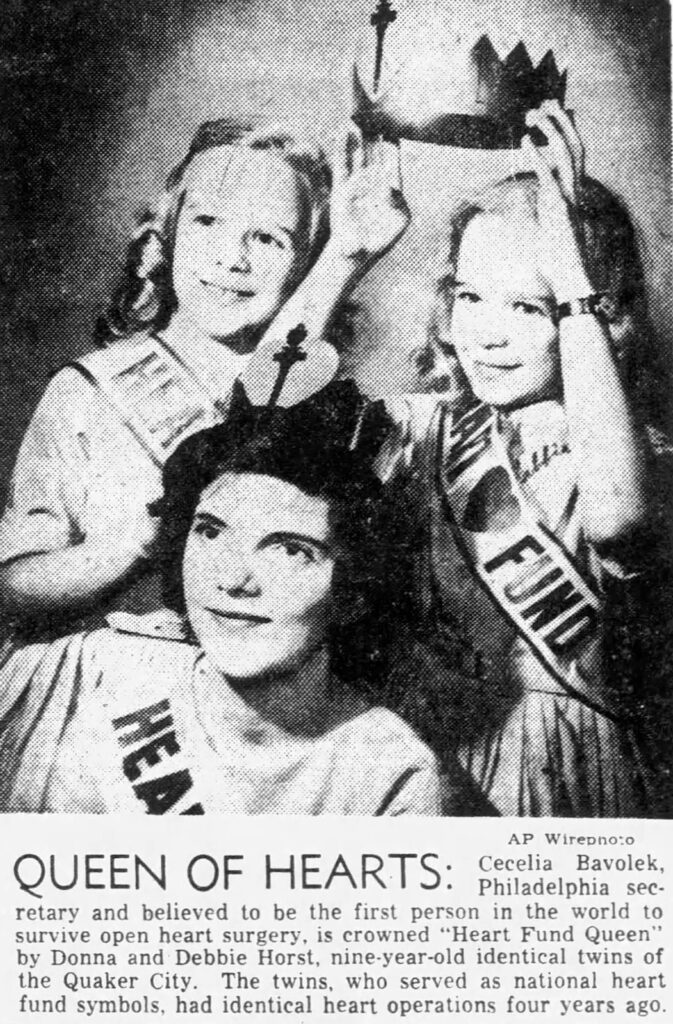
Maly, whom Jack often called his “greatest asset,” earned a master’s in social work and spent several years as a marriage counselor. She and the Gibbon children continued to feel that Jack’s patients always came first. None of the four kids grew up to become a doctor; Mary and John instead followed in their mother’s footsteps. Mary earned a master’s in social work and John earned a doctorate in psychology, despite his father’s misgivings. Jack had always believed that asking for help was a sign of weakness.
Like Bavolek, Jack Gibbon also disliked the attention foisted on him after her surgery. He maintained a fraught relationship with his invention, reluctant to be photographed alongside it and fearful that he had opened “some kind of Pandora’s box” that could put human life at risk—even if it offered hope where none had previously existed. He recoiled every time he opened a surgical journal to see open-heart surgery continuing to spread. After expanding surgery’s limits, he seemed to question what he had done.
He spent the years until his retirement in 1967 practicing surgery and writing regularly, including a book on chest surgery that he regularly updated. He was on the advisory board of Annals of Surgery until his death in 1973, from a heart attack suffered while playing tennis.
At a memorial service, Jefferson colleague Charles Fineberg acknowledged the sacrifice Jack’s work had demanded of others and the gulf between him and his children that he had never overcome.
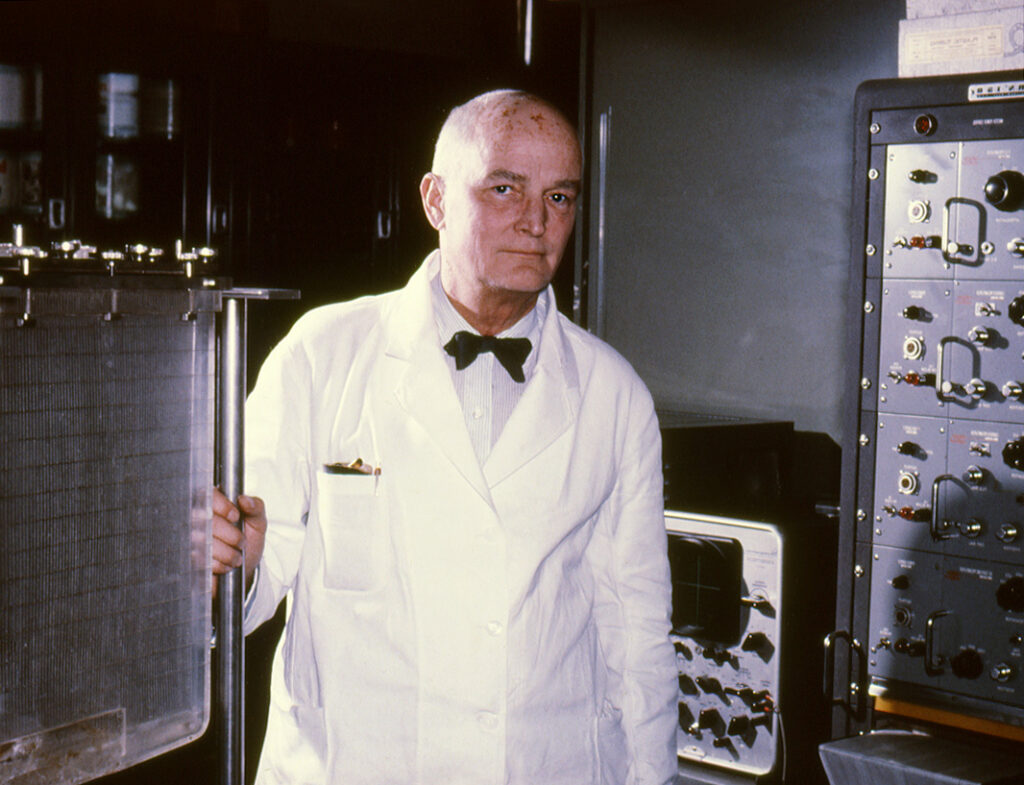
“For the years he gave to us, and took from you, we ask your forgiveness,” Fineberg said, acknowledging the toll Jack’s research had taken on the Gibbons’ family.
In 1955, in a presidential address to the American Surgical Association, Jack Gibbon spoke about the aspects of a surgeon’s education that he kept dear. His words read like those of a man who cared most of all about expanding the realm of possibility.
“We must throw away for good and all in our teaching, the concept that the world of knowledge consists of neatly ordered little boxes of facts,” he said. “We must point out that knowledge is dynamic, not static; that thoughtful men have ever continued to push back the curtains of darkness and throw more light on the mystery of life.”