In southern Peru, near the Pacific coast, powerful winds blow sand across high desert plateaus. The region is almost entirely barren except for ephemeral lomas, bright green oases sustained by fog banks that move with the weather. Every so often El Niño drowns the land with its relentless rain, turning sand into mud and washing away the few towns that exist in this arid landscape.
Southern Peru wasn’t always so desolate. More than 1,000 years ago the Chiribaya civilization thrived here by fishing along the coast; cultivating potatoes, maize, and other crops in river valleys; raising llamas as pack animals and cuyes (guinea pigs) as meat. Amid this bounty of resources the Chiribaya created pottery and textiles with intricate geometric designs and inscribed massive geoglyphs into hillsides with stone. They also practiced the art of mummification, just as other South American civilizations had done since 5000 BCE, around 2,000 years before the Egyptians started preserving their dead.
The relative affluence of the Chiribaya lasted for hundreds of years, until a series of invasions—ending with a conquest by the Inca Empire—nearly wiped out their culture. In the 1500s conflict and disease brought by Spanish conquistadors finished off the Chiribaya. The dry, dusty landscape has been only sparsely populated since. But a climate hostile to new settlement created ideal conditions for preserving the past. Archaeologists have discovered dozens of grave sites filled with pottery, jewelry, and well-preserved clothing and textiles. That level of preservation has long attracted looters and more recently anthropologists such as Sonia Guillén, a mummy expert who has picked through the remains for information about Chiribaya culture.
Between 1999 and 2002 Guillén and her colleagues discovered two mummified Chiribaya skulls while investigating the desert a few miles east of the port city Ilo. Looters had destroyed the bodies but had spared the heads, which were covered in braids of thick black hair. Because bones and clothing were missing, Guillén could not determine much about the individuals beyond when they lived. According to radiocarbon dating they probably died around 1025, almost 500 years before Christopher Columbus arrived in the Americas.
Radiocarbon Dating
Early paleontologists had few ways of determining the age of their discoveries. The best they could do was date fossils relative to other fossils based on the layer of rock they were found in. In the 1940s that finally changed when chemists Willard Libby and James Arnold developed a process they called radiocarbon dating.
Radiocarbon dating is an ingeniously simple idea that makes it possible to determine the age of bones and fossils with an accuracy of a hundred years or so. Through eating, drinking, breathing, or photosynthesis, all organisms absorb the radioactive isotope carbon-14, which is created from nitrogen-14 when cosmic radiation strikes the atmosphere. When an organism dies, it stops absorbing carbon, but the radioactive isotopes it has taken in through its life continue to decay at a consistent rate. Archaeologists can determine how long ago something lived by measuring how much carbon-14 is left. The less carbon-14, the older the object. Unfortunately, the method isn’t reliable for anything more than 55,000 years old because by that point almost all the carbon-14 has decayed. Archaeologists can use uranium and other radioactive elements to date even older remains but with less accurate results.
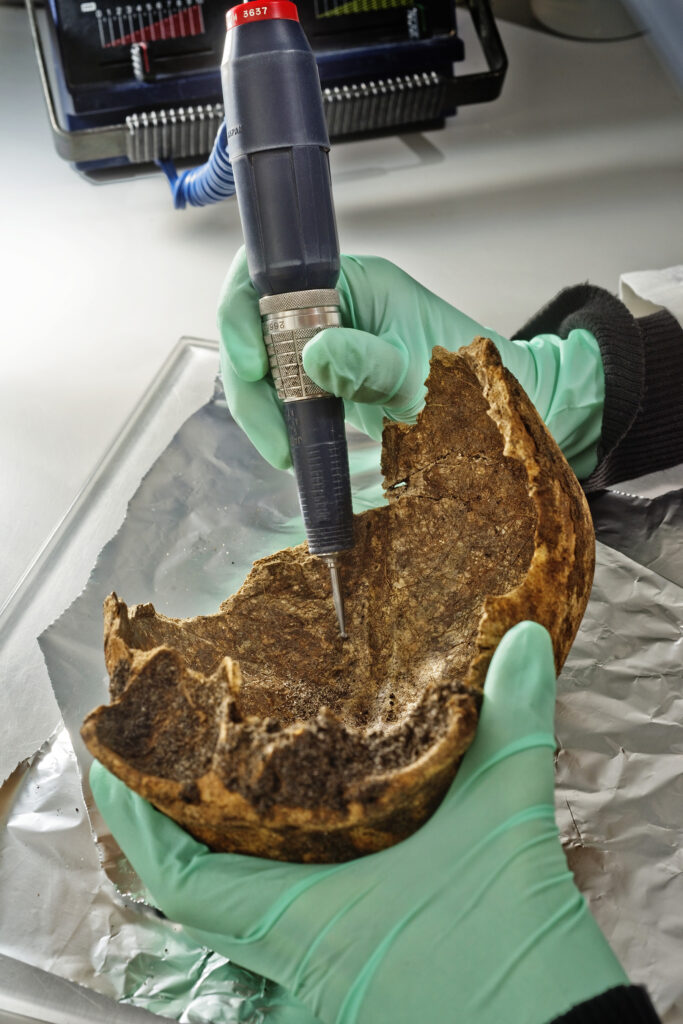
A University of Oxford researcher uses a rotary rasp to remove material from a Bronze Age human skull for radiocarbon dating.
James King-Holmes/Science Photo Library
Once the heads were removed from the cool, dry environs of their burial site in the desert, Guillén had to work fast to bring them to a climate-controlled laboratory before they decomposed. At some point during the transfer she noticed tiny stowaways in the mummies’ hair—lice that were unintentionally interred with the bodies. She bottled the lice up and eventually gave them to her friend Katharina Dittmar, a German evolutionary biologist who was then working on a postdoc about parasites. Dittmar incorporated the find into a 2003 paper describing which diseases might have plagued the Chiribaya culture.
A few years later Dittmar was working at the University of Buffalo when another evolutionary biologist named David Reed paid her a visit. Reed, then a postdoctoral researcher at the University of Utah, was familiar with Dittmar’s work and wanted to pick her brain about parasites. Dittmar mentioned that she had a vial full of hundreds of ancient, shriveled lice from Peru. Reed asked if he could try to sequence their DNA, and Dittmar handed over the vial.
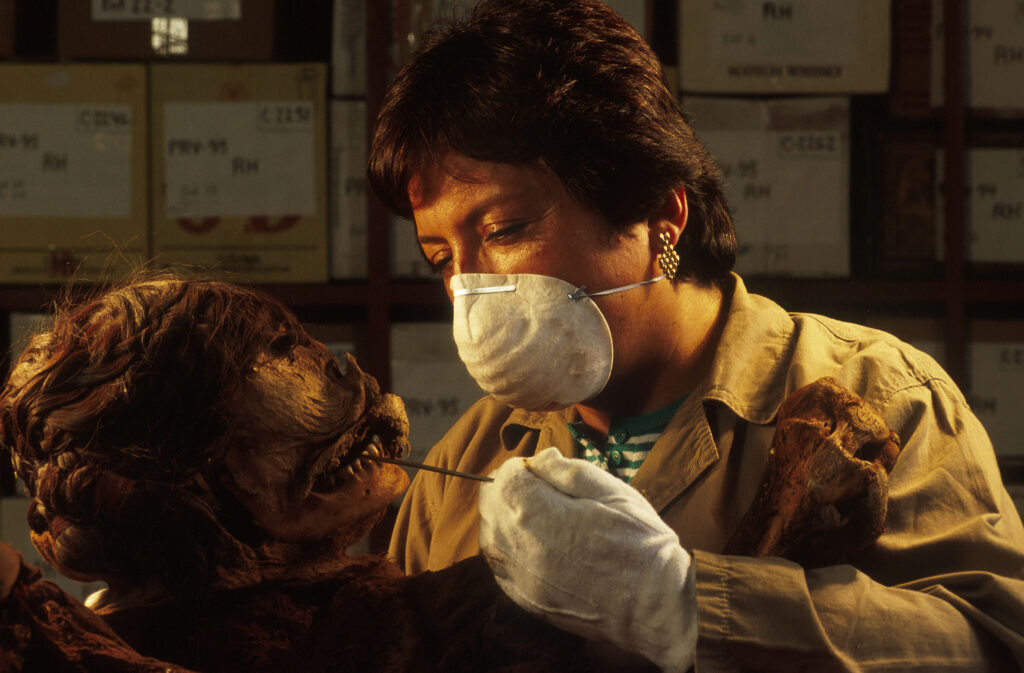
Peruvian anthropologist Sonia Guillén studies the teeth of a Chiribaya mummy in August 1998.
Reed, now associate director for research and collections at the Florida Museum of Natural History, has no special interest in lice; instead he uses them to study the early history of their hosts. Although we don’t know much for certain about the opaque time before our ancestors spread out of Africa or learned to make clothing, we do know one thing for sure: our ancestors were infested with lice, and wherever these early humans went, their lice went with them. Thanks to a quirk of the parasite-host relationship, tens of thousands of years later Reed wanted to use that knowledge to fill in gaps of human history. How did humans migrate across the planet? What events jump-started the spread of typhus and other parasite-borne diseases? These are questions Reed hoped to answer with lice.
Reed first learned about the unique nature of mammal-parasite relationships during graduate school at Louisiana State University. While analyzing parasites living on pocket gophers in New Mexico and Texas, he realized that each lineage of pocket gopher hosts its own unique lineage of louse. (A lineage of gopher or louse has enough genetic differences from its relatives to be distinct but not enough to be categorized as a new species.) Reed published a paper showing that these different lineages of lice are adapted to cling to the specific thickness of their hosts’ hair. Move a louse living on a gopher with thin hair to a gopher with thick hair, and the latter’s natural grooming behavior would brush the louse off; the groove on the louse’s head used to help cling to its host would be too small to attach to the wider hair.
Reed knew that lice had been living on pocket gophers for millions of years. He also knew that pocket gophers had evolved and changed during that time and that lice cannot survive long away from their hosts. Therefore, whatever happened to the gophers must also have caused changes in the lice; if the gophers’ hair grew thicker, so too did the groove the lice used to hold onto the hair. Mark Hafner, Reed’s adviser at LSU, called this process coevolution.
If lice coevolved with gophers, they must also have coevolved with other species they infest, including humans. That meant Reed could use lice to study the history of Homo sapiens, similar to how a climate scientist might use ancient pollen frozen in ice cores to learn about the planet’s environmental conditions thousands of years ago. But in this case the lice were the pollen and humans were their world.
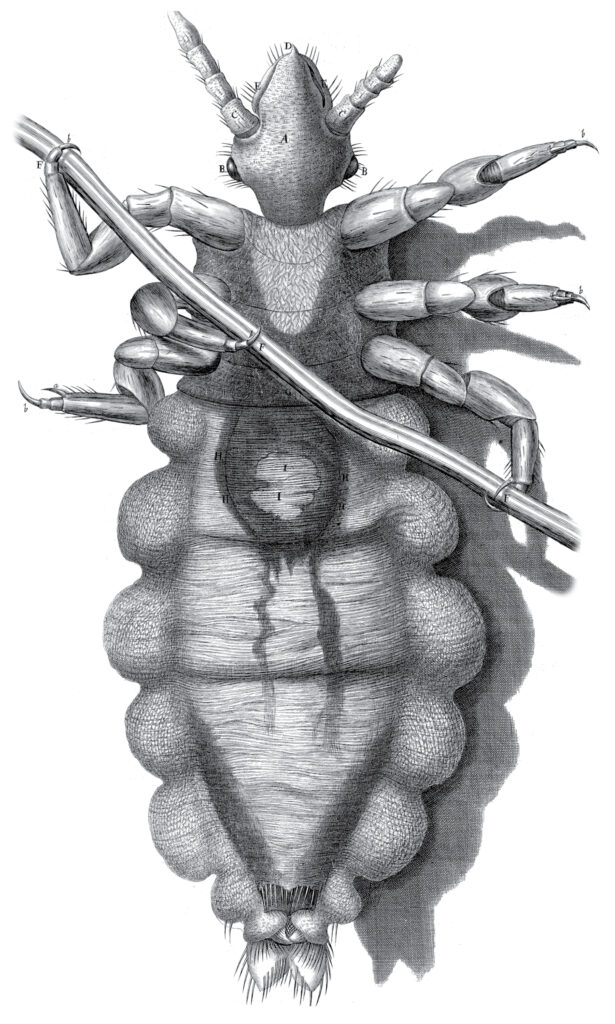
Robert Hooke’s famous rendering of a head louse, from Micrographia (1665).
Not long after completing his PhD, Reed moved to the University of Florida, where he started a database of louse DNA collected from all over the planet. But the Peruvian head lice represented his first chance to study louse DNA from the past.
At first Reed wasn’t sure he would get anything useful out of the mummified lice. His equipment was designed to extract DNA from fresh lice, and he had no idea of the condition of the 1,000-year-old specimens. Yet shortly after he dipped them in an alcohol solution to reconstitute them, he marveled that “they looked like they were collected yesterday.”
Reed could easily identify six hairy legs sprouting from flat abdomens about the size of a sesame seed. The desert climate had dried them out so quickly after death that their exoskeletons shrank, acting as protective pouches for their soft innards and DNA. Essentially, their insides had been hermetically sealed by the dry air.
The Human-Louse Connection
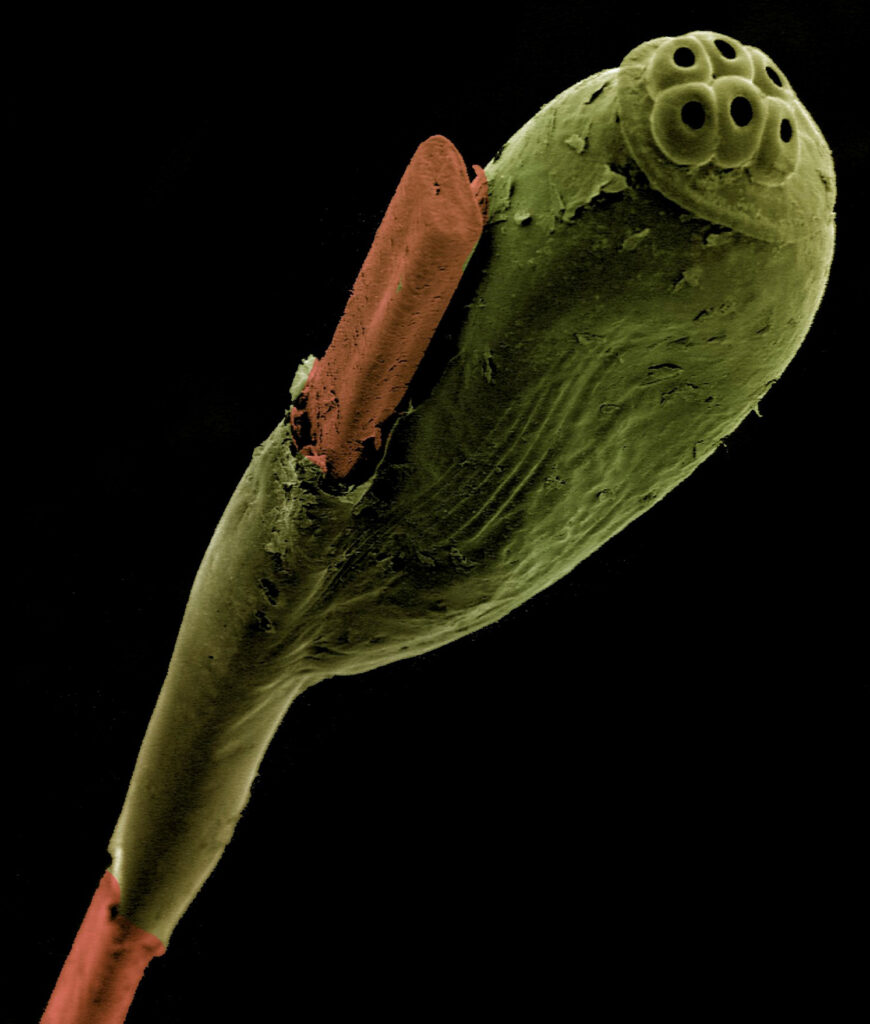
A colorized scanning electron micrograph of a head louse’s egg attached to a strand of human hair.
Humans are unique in that we host three forms of lice. The head louse is most recognizable: it’s the bane of schoolchildren around the world. Head lice have small claws that are perfectly shaped to allow them to climb up and down shafts of our hair, where they lay eggs. Several times a day they have to descend from their perch to feed on the scalp of their host. European men in the 1700s shaved their heads and wore powdered wigs in part to combat the pests. However, they shaved their heads not to thwart lice, but rather to better fit the wigs, which were periodically boiled and brushed to remove the lice.
The second kind of louse that makes a home on humans is the clothing louse (also known as the body louse). These insects are a little larger than head lice and lay their eggs in practically every kind of fabric humans come into contact with. Clothing lice are also the only type of human lice known to spread bacterial diseases, such as typhus and trench fever, and are most often a problem for refugees and homeless people who don’t have opportunities to wash their clothes and bedding regularly.
The third type of louse lives primarily in the pubic region and is spread through sexual contact. Resembling bulky crabs with huge claws designed to grip thick hair, their origin is perhaps the most perplexing of all three species: millions of years ago our forebears inherited them from the ancestors of gorillas.
Reed carefully sliced them in half and extracted and analyzed their DNA. Then he compared it to the genetic data he had collected from modern lice all over the world. This is where things get a little bit complicated.
There are three types of lice that live on humans: head, clothing, and pubic lice. These parasites are divided into a handful of major genetic groups, or clades. Perplexingly, these clades do not line up with the head, clothing, and pubic lice categories. Although there are at least five clades, only two of them are widespread enough to be relevant to this story. Clade A lice are common on every continent and consist of both head and clothing lice. Clade B lice are limited to living on human heads and originated in the Western Hemisphere, but have since spread to Europe and Australia. These categories can be difficult to keep straight. It might be helpful to think of clade A as global lice (everybody has them and they live all over the body) and clade B as American lice that prefer the head.
At some point in history each clade became separated from the others for long enough to develop its own unique DNA. (Think of finches in the Galapagos and how their beaks evolved to harvest the food available on their respective islands.) Yet for lice the differences in their genes that define the different clades are not evident at a glance and can only be detected by sophisticated computer programs that find patterns over entire genomes.
Although clades A and B are currently found in the Western Hemisphere, Reed knew they were so genetically distinct that they must have diverged tens of thousands of years ago. Where did clades A and B come from, and which one arrived in the Americas first? Reed hoped the answer to that question might help resolve disputes over how and when humans migrated to the Western Hemisphere.
Back in the early 2000s, while Guillén was digging around ancient tombs in the Peruvian desert, Reed was finishing up his postdoctoral work and deciphering the evolutionary fingerprints of louse DNA. Using a computer program that calculated the rate of mutations and change between the two species, he concluded that the two main clades of lice, A and B, had diverged 1.18 million years ago. Reed was perplexed: how could a species as young as humans (our current anatomical form has been around for less than 300,000 years) have such ancient lice?
A 2003 article in Nature summarizing the understanding of human evolution at the time gave Reed an idea of where the ancient lice could have come from. In particular, a graphic within the article jumped out at him. It showed a proposed split between Homo erectus and the ancestors of H. sapiens that very roughly corresponded to the split in lice clades he calculated to have occurred 1.18 million years ago. That meant some major event must have happened at that time, causing two populations of hominins (a term that broadly encompasses humans and our close, extinct relatives) to be separated for more than a million years and leading their lice to evolve distinct genetic traits.
Reed theorized that the major event could have been when H. erectus began the process of migrating out of Africa, leaving behind the direct ancestors of H. sapiens. That gave Reed another idea. What if clade B lice didn’t belong to H. sapiens at all but originated on a population of H. erectus that had left Africa? It would account for the isolated populations in the Western Hemisphere because at the time the fossil record showed that H. sapiens didn’t leave Africa for another million years.
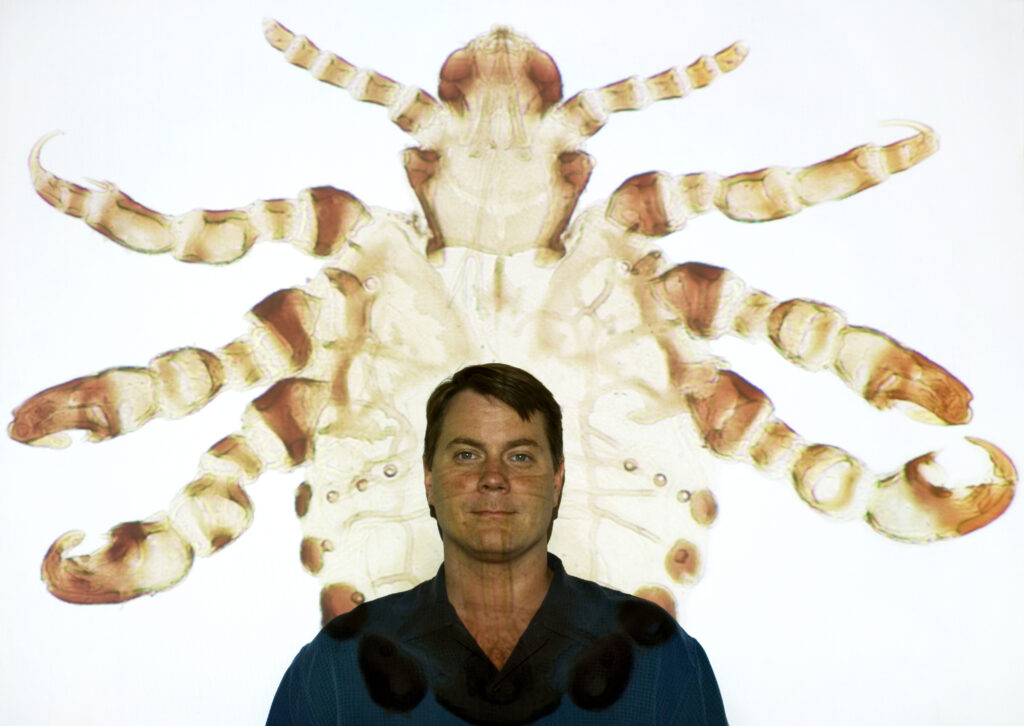
Evolutionary biologist David Reed.
But Reed’s theory raised an obvious question: how would H. erectus’s clade B lice—which are native to the Western Hemisphere—have hitchhiked to the Americas? There is no evidence that H. erectus ever made it to the Western Hemisphere. Reed’s explanation was simple: after H. sapiens left Africa 100,000 years ago, some populations met H. erectus in Asia. The two species swapped lice before H. erectus went extinct; H. sapiens then continued on with its new parasites to the New World.
For this theory to make sense H. sapiens and H. erectus must have been in close contact for a while, long enough to allow their lice to exchange genetic material. That meant they were living with each other and maybe even mating, a possibility that had not been previously considered.
Reed published his proposal in 2004 in the journal PLOS Biology to a mixed reception. Mark Stoneking, a geneticist at the Max Planck Institute for Evolutionary Anthropology, complained to the New Scientist that he had “serious reservations” about Reed’s conclusions. “While contact between modern and archaic humans is still a possible explanation for their results,” he said, “other explanations are more likely in my view.” Similarly, paleoanthropologist Milford Wolpoff told Science that Reed’s paper was nothing more than a “fringe explanation.”
A Brief Overview of Early Human Evolution and Migration
It seems like every few months scientists find new fossil evidence of ancient humans that changes our understanding of the past (and makes articles like this one outdated). It’s a little ironic that the field of archaeology, which studies imperceptibly slow changes over a large enough timescale to make them perceptible, is roiled so frequently by changes itself. Although new discoveries are piling up faster than scientists can parse them, the big question of exactly where humans came from and why we succeeded over other species remains a mystery. Still, scientists have a broad outline that describes what probably transpired.
The story goes that somewhere in Africa at least 7 million years ago, a series of random genetic mutations began to cause a split between our ancestors and other primates. (This was a messy break that took millions of years to complete and had multiple false starts.) While ancient chimpanzees and bonobos stuck to the jungle, archaic humans left the trees for the grasslands where their bodies and brains adapted to a more terrestrial lifestyle.
Some of the earliest fossil evidence from this period comes from Homo habilis, an archaic species that lived 2.4 million to 1.4 million years ago. H. habilis was short in stature and had long arms and a relatively small brain. Some members of H. habilis evolved into a new species called H. erectus, an adept tool user with a considerably larger brain capacity, that expanded out of Africa and went on to settle most of Asia about 1.3 million to 1.8 million years ago. The muscular H. neanderthalensis, the Neanderthals, branched off from H. erectus about 200,000 to 400,000 years ago and colonized Europe, where they adapted to withstand the colder temperatures.
H. sapiens is related to all these species but likely descended most directly from the group of H. erectus that stayed behind in Africa. (Other theories exist, though, most notably that H. sapiens comes from a lineage of H. erectus located in Asia.) When a species of plant or animal diverges from its ancestors, the older species doesn’t cease to exist; both organisms continue evolving in tandem until the less successful one dies off or splits again. In this case H. erectus did disappear from Africa while our direct ancestors thrived, although no one knows exactly how H. sapiens came to be.
Beginning roughly 120,000 years ago, more-or-less anatomically modern H. sapiens began leaving Africa in waves, perhaps forced out by a drought or by following the migrations of game animals. Genetic evidence indicates that all human beings on Earth are descended from just one of these waves, a relatively tiny population, that happened to be the most successful. These explorers traveled into the Middle East and then split up; some went to Europe while others colonized Asia over the course of thousands of years. About 65,000 years ago at least one group migrated into Australia; another group crossed a temporary land bridge to North America as early as 30,000 years ago (where some of their descendants ultimately settled in the Peruvian desert).
Wherever H. sapiens went, other large mammals went extinct, including H. erectus and the Neanderthals. H. erectus died out around 30,000 years ago, and the Neanderthals began to vanish around the same time. Eventually the only human species left was H. sapiens. We had inadvertently taken over the world.
Of course evolution is never linear. Most of these archaic humans interacted and evolved alongside one another, fighting and likely breeding when they came into contact. Although they undoubtedly had different cultures and body structures, they all shared one thing: lice.
So when Reed analyzed Guillén’s mummified lice in Florida four years later, they provided more than just an opportunity for him to learn about humanity’s past. They had the potential to be the evidence that would quiet his naysayers. If these pre-Columbian lice belonged to clade B, as he suspected, that would mean the populations of H. sapiens who settled in North America 15,000 years ago unquestionably brought a unique genetic lineage of lice to the Western Hemisphere before anyone else, lice they could have picked up from H. erectus in Asia during their migration. If Reed was right, the lowly louse would permanently change our understanding of human history.
But Reed was wrong. His analysis showed the Peruvian lice belonged to clade A, not clade B. A laboratory in France duplicated his results. Somehow, clade A lice ended up in South America before European settlers arrived with their own clade A lice. While the findings torpedoed his theory, Reed realized this new discovery had compelling implications of its own.
First, it added evidence to the idea that one wildly successful wave of H. sapiens, who left Africa all at once, colonized the entire world by themselves. It was the only logical explanation for how the exact same kind of lice could be on the bodies and heads of people all the way from Australia to Norway before global trade began.
Second, Reed realized his new findings might help reevaluate a topic scientists had long considered settled: the geographical source of typhus.
In the past 500 years the epidemic typhus bacterium, Rickettsia prowazekii, has shown up in the wake of war, famine, and mass migration, most notably when it killed millions of soldiers and civilians in Eastern Europe during World War I and after the Russian Revolution of 1917. Epidemic typhus—not to be confused with typhoid, which is transmitted through contaminated food and water, or endemic typhus, which is transmitted by fleas—is spread by infected clothing lice, which thrive among groups of people crammed into unhygienic, crowded conditions. If left untreated, the disease can progress from symptoms such as rashes, headaches, and vomiting all the way to pneumonia, kidney failure, and the collapse of blood vessels in the body.
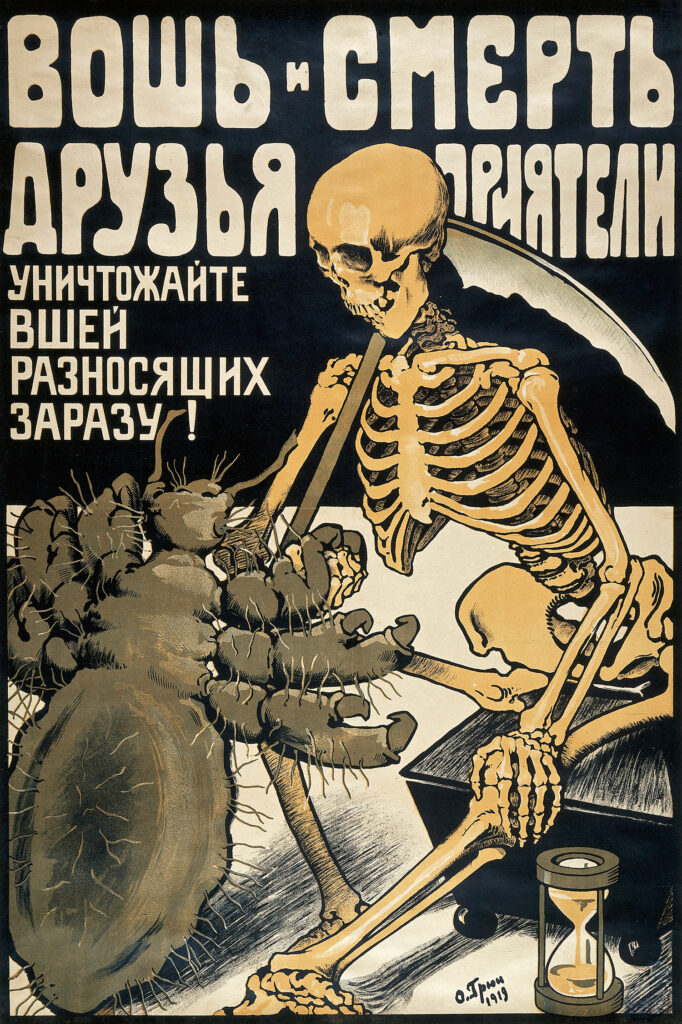
A typhus louse and Death shake hands in this Russian poster from 1919. The text translates as “The louse and death are friends and comrades. Kill all lice carrying infection!”
After Europeans made landfall in South America in 1498 but long before those explorers reached the Chiribaya’s home in what is now Peru, they unwittingly unleashed a number of insidious weapons. Smallpox, yellow fever, malaria, and other diseases sped ahead of the European invasion, carried in the bodies of fleeing refugees and in the guts of swarming mosquitoes. By the time conquistadors reached the Inca cities of Cusco and Chan Chan, millions had died or abandoned their homes.
For a long time scholars logically assumed that epidemic typhus was just another illness brought by Europeans to the New World. After all, it was the indigenous Americans who were killed by the disease in droves during the conquest, not European invaders. However, Reed’s research showed that the currently known vector for typhus, clade A clothing lice, was present in the Americas before the Europeans got there. What’s more, the few records of “typhus” in the Old World before the 1500s could have been describing other diseases with similar symptoms. It wasn’t until after the invasion of the Americas that the disease popped up in European military camps and prisons, killing hundreds of thousands. Theoretically, the clade A lice living on indigenous Americans could have passed the bacterium to European lice. Could typhus be a rare example of a disease traveling from west to east across the Atlantic, a small story of payback to the invaders?
It’s a compelling question, but for now it remains unanswered. Other researchers, including French biologist Didier Raoult, had already published papers examining the possibility before Reed weighed in. However, Raoult concluded that it would be extremely difficult to find a definitive answer. Reed himself has suggested that if a natural reservoir for typhus was identified in the Americas—say, in the lice living on flying squirrels—then the theory would carry real weight, but he is not currently pursuing the origin of typhus. Still, there would be practical value in such research: no widely available vaccine exists for typhus (although it is treatable with antibiotics), and understanding the origin of the disease might help researchers find better ways to treat it.
The same clothing lice that carry typhus offered Reed one more insight into humanity’s past. Shortly after publishing his paper on the mummified Peruvian lice in 2008, Reed was reminded of an older paper published in 2003 by Mark Stoneking, one of the detractors of Reed’s H. erectus theory. In his paper Stoneking argued that because organic clothing is not preserved in the fossil record, it would be nearly impossible to find physical evidence of the first time humans wore clothes. Stoneking also pointed out that clothing lice can’t survive long away from clothing; they are perfectly adapted to living in fabric close to the human body, complete with hooked claws that keep them from falling off. In other words they couldn’t have existed before humans started wearing clothes. If you find the origin of clothing lice, you find a very rough date for the origin of human clothing.
Using computer models of louse DNA, similar in method to the one Reed used to differentiate between clade A and clade B lice, Stone-king determined that clothing lice diverged from head lice between 30,000 and 114,000 years ago, meaning humans were wearing clothes by then. One theory suggests that humans lost their body hair in part to combat parasites, forcing lice to abandon our bodies and to respecialize in living on people’s heads. After humans started wearing clothes, some of those head lice evolved again and migrated south to take advantage of the new threads.
Reed was intrigued by Stoneking’s research but was unimpressed with his methods, even suggesting that Stoneking had incorrectly calibrated the “molecular clock” he used to determine how long ago clothing lice diverged from head lice (see sidebar). The problem, it turned out, was that Stoneking had decided to use DNA from a chimpanzee louse to calibrate the rate of divergence in his computer model. That would have been fine if he had actually used chimpanzee louse DNA. Instead, he had accidently used a DNA sequence from another species.
When Reed redid the analysis, he made sure he was using actual chimpanzee louse DNA and came to a different conclusion. Reed determined that lice left our heads and evolved into clothing lice as early as 170,000 years ago. That was long before fossil evidence suggests that H. sapiens finished migrating out of Africa, meaning our ancestors may not have developed clothes in response to migrating to colder climates and that they lost their body hair sometime before they left. In 2011 he published a paper on his findings gently correcting Stoneking’s results.
The Molecular Clock
In 1962 biologist Emile Zuckerkandl and chemist Linus Pauling proposed an idea that would allow scientists to look into an organism’s past using only its DNA and a handful of other molecules; they called their concept the molecular evolutionary clock. Some scientists were skeptical. How could a living organism provide useful information about its ancestors?
Zuckerkandl and Pauling suggested that if you know the rate at which an organism accumulates molecular variations (mutations, more or less) over time, you can figure out how long ago that organism’s ancestors diverged from the original species (for example, when one species of louse turned into two). The molecular clock has been explained in various ways over the years, but Al Tanner, a data scientist formerly with the Bristol Palaeobiology Research Group, may have done it best.
Imagine a book inside a monastery’s library, Tanner suggests. As the book ages and falls apart, monks preserve it by transcribing it by hand. But each time a monk copies the book, he makes a small mistake—an accidental misspelling or a forgotten period. Changes accumulate, but they are small and incremental, so they go unnoticed.
Eventually, a monk gives a copy of the book to another monastery so others can read it. Monks at the new monastery begin copying it and introduce errors of their own over many generations.
Much later a researcher discovers the latest versions of the book at each of the two monasteries. The books are slightly different after being transcribed in isolation for so long, but the researcher realizes they must be based on the same original text and wants to know when they diverged. After comparing the books the researcher finds an average of 100 differences per page. Having studied many other ancient books, the researcher knows that the monks probably made one mistake every 10 years. Using a simple calculation the researcher concludes the book was brought to the second monastery around 500 years ago: 100 differences per page, multiplied by the 10 years each mistake took to show up, divided by two versions of the book.
When David Reed wanted to determine when clade A lice split from clade B lice, he used this process.
Although the study of lice does not directly answer why humans started wearing clothes, whether our ancestors might have gotten intimate with H. erectus, or how two different clades of lice ended up spread around the world, they do offer concrete dates that Reed, Stoneking, and other archaeologists and anthropologists can use to make their own research more accurate.
For decades geneticists have suspected that modern humans interbred with our extinct relatives, and although Reed’s idea that H. erectus and H. sapiens could have interbred along with their lice proved to be unsupported, he was one of the first scientists to look for concrete answers.
“Since we did this work, scientists know much more about both the lice of humans and humans themselves,” Reed says. “For example, there are now five to six different groups of lice, and they likely evolved on multiple species of now-extinct hominins that lived at the same time. In other words, we learned that what really happened is more complex than we appreciated at the time we started this research.”
In just the last few years the technology to extract and compare Neanderthal DNA to that of modern humans on a large scale has finally become feasible. In 2010 a study found that the genomes of most humans appear to consist of 1% to 4% Neanderthal DNA. If lice are ever found on the head of a mummified Neanderthal, we might be able to figure out exactly where and when that breeding occurred.