The world is not likely to run out of magnesium anytime soon. As the eighth most abundant element on Earth, the metal makes up roughly 2% of the planet’s crust. It’s also the third most plentiful element in seawater, but unlike other metals, such as gold or copper, it only exists in nature in combination with other elements.
Magnesium’s initial discovery is credited to Scottish chemist Joseph Black, who performed a series of experiments with magnesium carbonate in the 1750s. Humphry Davy first reported magnesia was the oxide of a new metal in 1808, but it would take 20 more years before French scientist Antoine Bussy obtained magnesium in its pure metallic form.
Production of magnesium on a commercial scale started in Germany in 1886. Commercial magnesium production was made possible by using a modification of an electrolytic cell developed by Robert Bunsen. Magnesium chloride would be heated to between 655°C and 720°C (or roughly 1200°F to 1325°F), then zapped with an electrical charge to separate the compound into molten magnesium and chlorine gas. This process, the principles of which are still used today, allowed Germany to dominate the early magnesium market, which at the time was still relatively small.
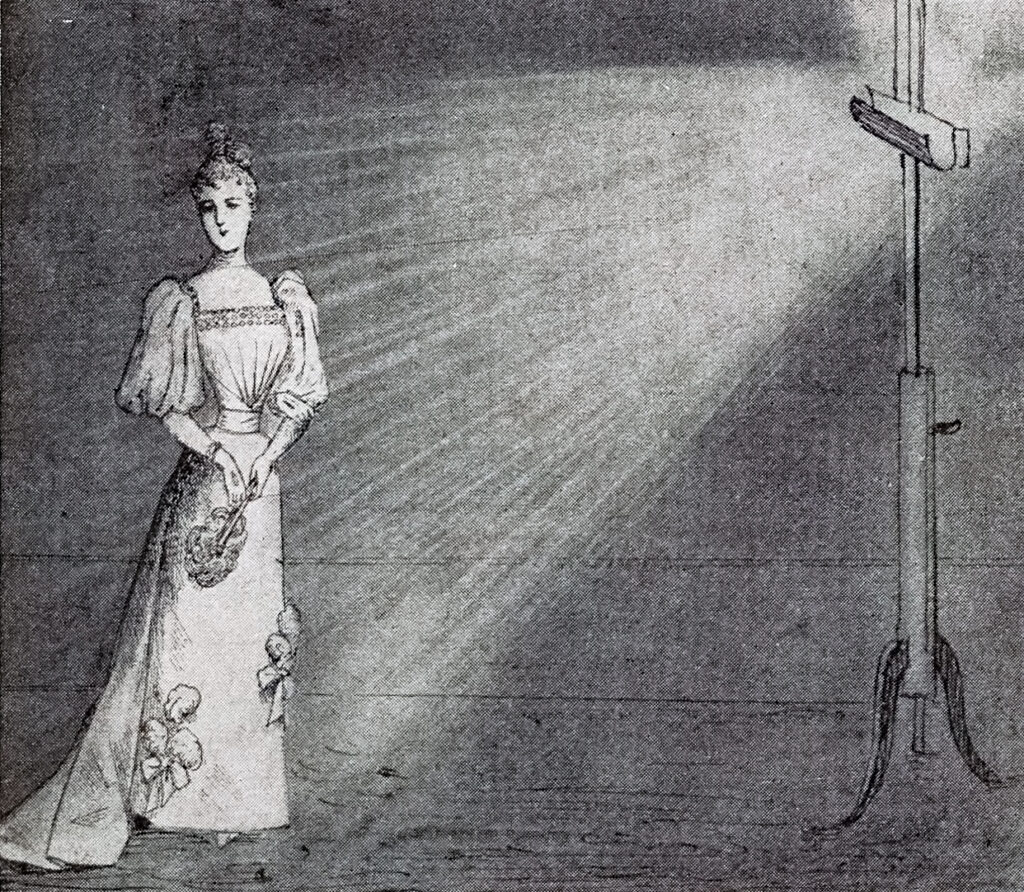
Illustration of a magnesium flash in action from Ris-Paquot’s La pratique de la photographie à la lumière artificielle.
Initially, magnesium proved useful for triggering many laboratory reactions, especially organic reactions, where it is used as a Grignard reagent. As an essential element for growth in human organisms, it was also added to many foods and fertilizers. Outside of the laboratory, magnesium was used almost exclusively for its illuminating properties, particularly as a flashbulb in photography. Although uses for the metal were initially limited, demand boomed with the outbreak of war.
Magnesium found many uses in the trenches of Europe during World War I, particularly in the aptly named “star shells,” which were used to light up the battlefield at night. The element was also used in tracer bullets, flares, and incendiaries. Yet production outside of Germany remained small and sporadic until the British navy’s blockade of Germany forced American manufacturers into the business. Herbert H. Dow, founder of the Dow Chemical Company in Midland, Michigan, was quick to answer the call.
As it happened, the briny, prehistoric sea that lay under Midland was flush with magnesium chloride, among many other chemicals. Dow had already figured out a way to efficiently extract bromine and chlorine from this brine and in the process had laid the foundation of his chemical empire. But extracting and selling chemicals from the brine was one thing; fabricating metals was something else entirely.
When the British blockade cut off German imports, the resulting spike in magnesium prices gave Dow an opportunity to enter the market. In 1916, after a period of trial and error, Dow employees produced the first ingot of magnesium. Although it was only a small cake from an experimental electrolytic cell, it was a sign of much bigger things to come. After a period of research and development, the company scaled up operations and sold a modest 3,852 pounds of magnesium in 1918, with nearly all of it going to the war effort.
After the war, demand for magnesium faded rapidly, and the ability to produce and sell the metal at a profit caused all but two U.S. companies to leave the industry. Several years later only Dow remained.
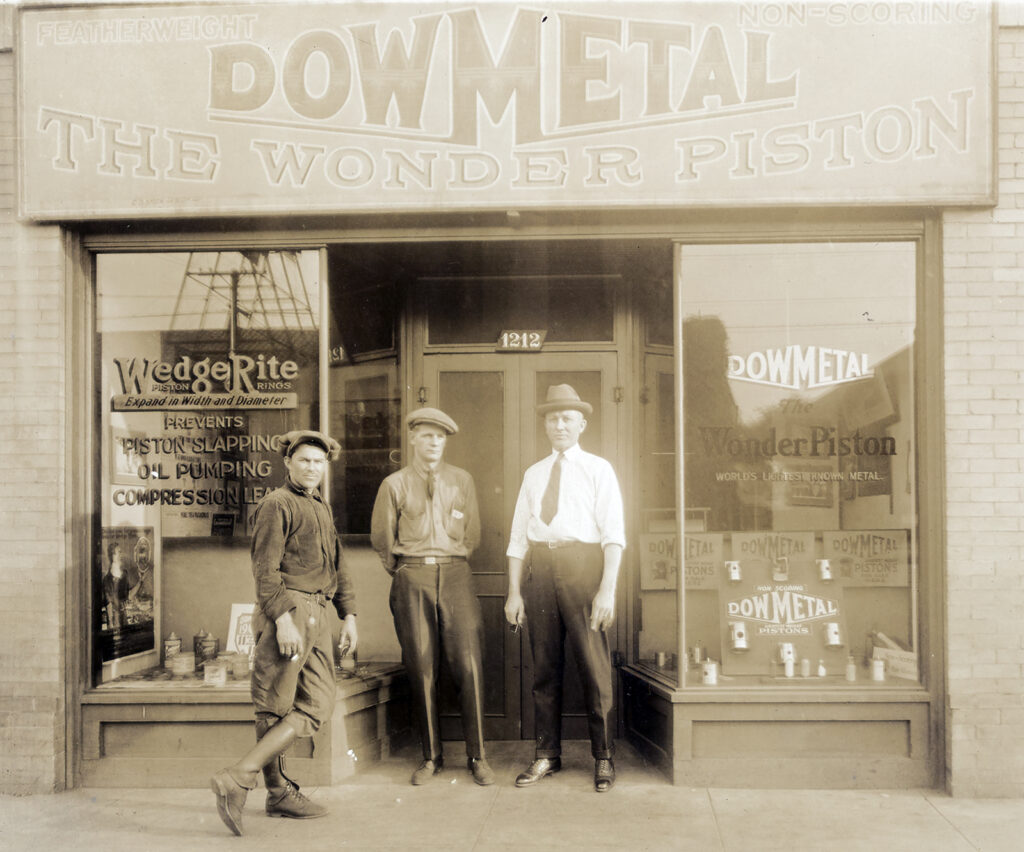
An store advertising automotive equipment, include pistons made from Dow magnesium, ca. 1920s.
Herbert Dow was convinced that with sufficient R&D, magnesium’s potential for structural applications was great. Magnesium is extremely lightweight when compared with many other metals, but it lacks sufficient strength in its pure state for most structural uses. By alloying magnesium with small amounts of other metals, such as aluminum, zinc, and manganese, the company found it could create alloys that were stronger than magnesium alone while maintaining magnesium’s lightweight characteristics and solving many of magnesium’s inherent corrosion problems.
But in those early days, industry showed little enthusiasm for Dow’s new product. Aluminum was the structural metal of choice, and if Dow wanted to expand its magnesium business, it would have to create the market itself.
When Herbert Dow learned the nearby General Motors Company intended to switch to aluminum pistons in its engines because they were lighter and thus required less power to operate, he saw an opportunity to introduce magnesium to the automobile industry. Replacing pistons in automobiles was common at the time, and Dow assumed that if aluminum pistons were desirable for their light weight and strength, then magnesium pistons would be even more desirable. He set up a separate department at Dow to manufacture magnesium alloys, which would be marketed as Dowmetal. The magnesium pistons cost more than those made from other alloys, and that hampered their sales, but they soon became the preferred choice for automobile racers looking for an edge. These “racing pistons” were used in many winning race cars during the early 1920s, including the Frontenac driven by Tommy Milton at the 1921 Indy 500.
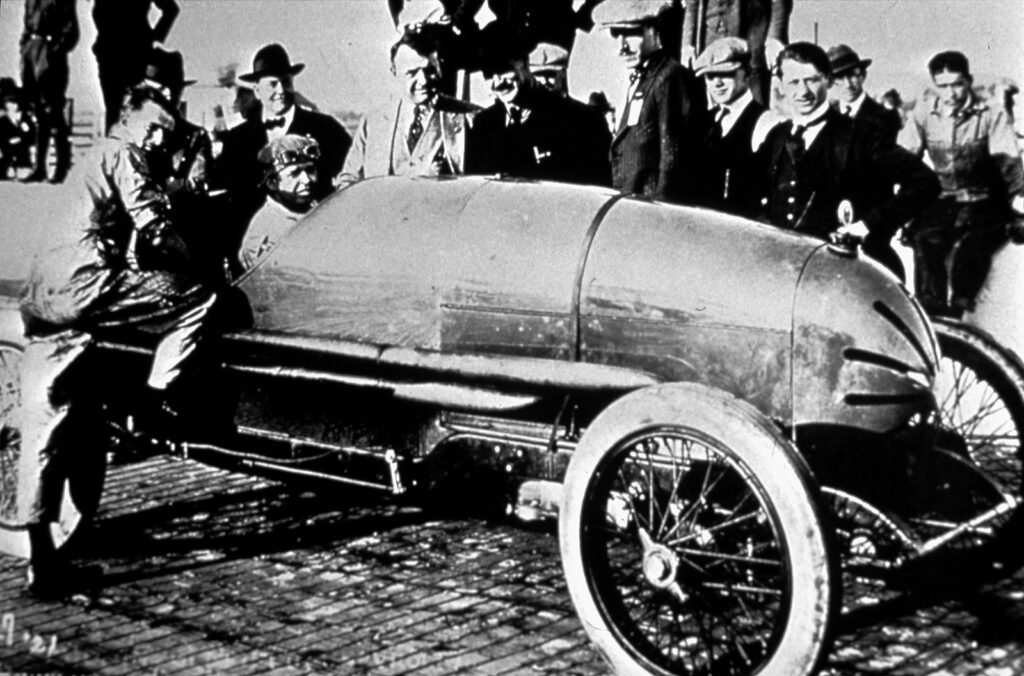
Tommy Milton and the Frontenac he used to win the Indianapolis 500 in 1921. The car featured Dow’s magnesium pistons.
The replacement piston business was well established by the mid-1920s when Dow enticed the aircraft industry to begin using magnesium in aircraft engines, landing-gear parts, seats, wheels, and various other applications. Magnesium proved reliable in these settings and soon was introduced in machinery parts, hand tools, and vacuum cleaners, to name just a few.
One of the more memorable applications of magnesium was its use in three gondolas used to carry passengers in a series of stratospheric balloon flights between 1933 and 1935. The first such gondola was built in Midland and was designed by famed balloonist Jean Piccard for the Chicago World’s Fair.
Earlier iterations of the gondola had been produced from aluminum, but it was hoped that the weight saved by using magnesium would allow for a new altitude record. Dow jumped at this golden marketing opportunity for Dowmetal and agreed to build the gondola at the company’s expense.
Thomas G. W. “Tex” Settle of the U.S. Navy was the lone crew member for the flight, but 20 minutes after lift-off a faulty hydrogen valve forced him to crash-land the balloon. Settle, however, was undeterred, and after the gondola was repaired, he lifted off again in November 1933, this time joined by Chester Fordney of the U.S. Marine Corps. The balloon, which took off from Akron, Ohio, floated at peak altitude for two hours before landing gently in Bridgeton, New Jersey. When the equipment they were carrying was examined by the National Bureau of Standards, it was confirmed that they had climbed a record-breaking 61,237 feet (11.6 miles).
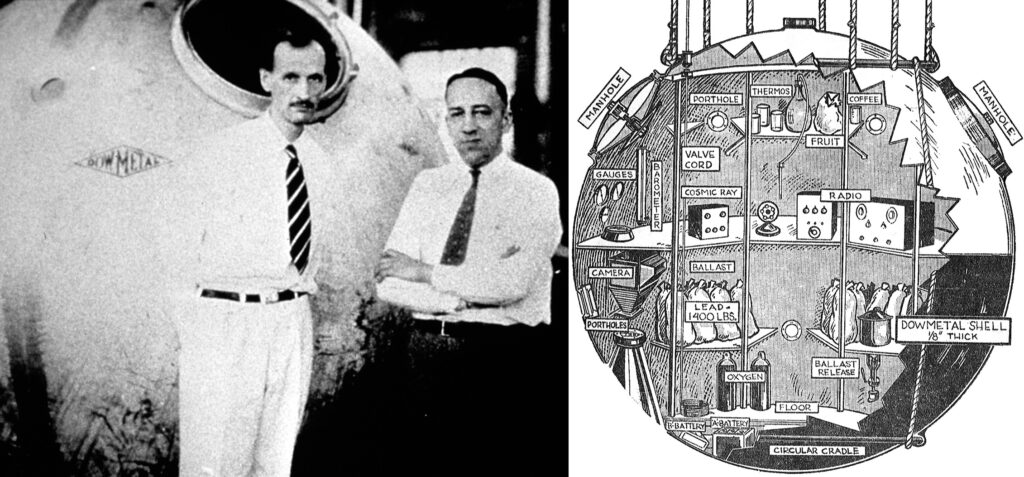
Left, Jean Piccard (left) and Tex Settle in front of the magnesium gondola used on Explorer, ca. 1933. Right, Chicago Daily News illustration depicting the instruments and supplies aboard Explorer, August 1933.
The following year, the Army Air Corps and the National Geographic Society teamed up to plan another recording-setting expedition into the stratosphere using a redesigned magnesium gondola named Explorer. The gondola weighed just 700 pounds, far less than the ship’s massive balloon bag, which tipped the scales at nearly two and half tons and required 1,500 cylinders of hydrogen gas to fill to capacity.
On July 28, 1934, three Army Air Corps officers lifted off from a site near Rapid City, South Dakota, and soared to within 624 feet of a new record, when a sudden rip in the balloon fabric caused a hydrogen-gas explosion and sent the craft plummeting back to Earth. All three men managed to parachute to safety, but the gondola was destroyed. The expedition planners were determined, though, and began plans for a second expedition in 1935.
On November 11, 1935, the third magnesium gondola, Explorer II, set off from Rapid City. This time the balloon was filled with helium to prevent another explosion, but since helium lacks the lifting power of hydrogen, the ship required an even larger balloon bag. The Goodyear-Zeppelin company used three acres of cotton fabric to create a balloon that could hold 3.7 million cubic feet of helium.
The new ship also sported a redesigned gondola that was both larger and lighter than its predecessor. It was painted black on the bottom to absorb heat from the earth and white on top to reflect the sun’s intense rays. Manning the ship were Orvil Anderson and Albert Stevens, both of whom had narrowly escaped death in the crash of the Explorer in 1934.
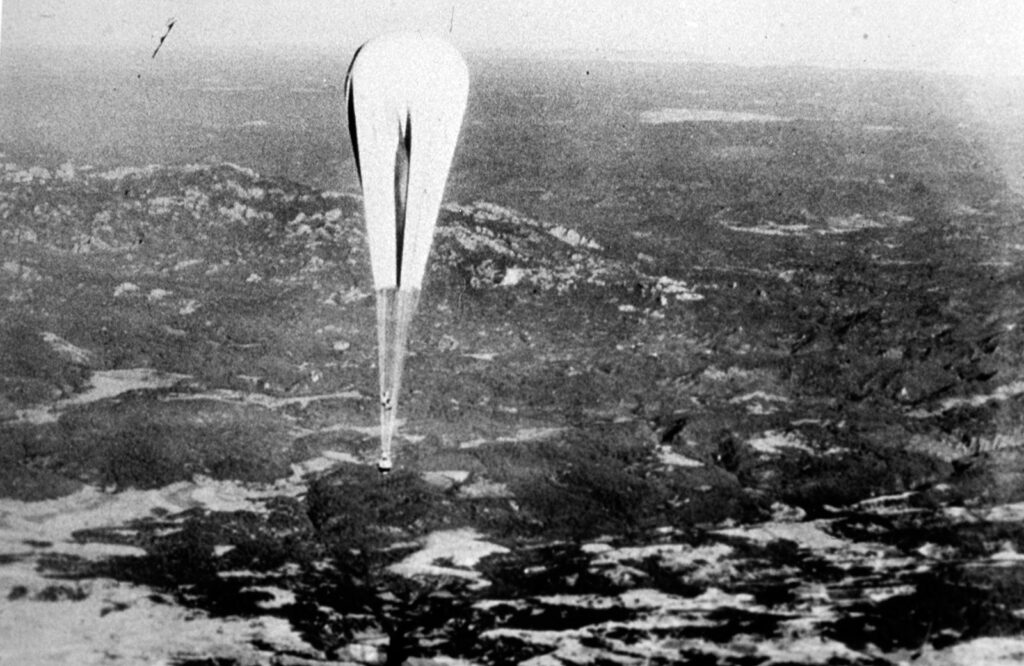
Photograph of Explorer II in flight, November 1935.
Almost four hours after lift-off, Explorer II reached an altitude of 72,395 feet (13.71 miles), setting a world record that would last for 21 years. At that height nearly 96% of the atmosphere’s mass was below the balloon, allowing Anderson, Stevens, and the 64 different scientific instruments on board to conduct an unprecedented study. The crew gathered new information on the nature of cosmic rays, the distribution of ozone in the upper atmosphere, the brightness of the sun, and the chemical composition of air above 70,000 feet. The eight-and-a-half-hour flight captured the imagination of people around the world.
Information gained from these flights was later used during World War II to give American airmen superiority in the skies over Europe and the Pacific. Advances to magnesium alloys, pressurization techniques, and personal equipment, such as heated flying suits and two-way radios, all came out of these expeditions. By 1958 that information began bearing further fruit in the U.S. space program.
While the magnesium used in the gondolas was extracted from Michigan brine, it was becoming increasingly apparent that the Midland wells would be unable to fulfill the growing demand for magnesium, bromine, and the many other chemicals Dow produced.
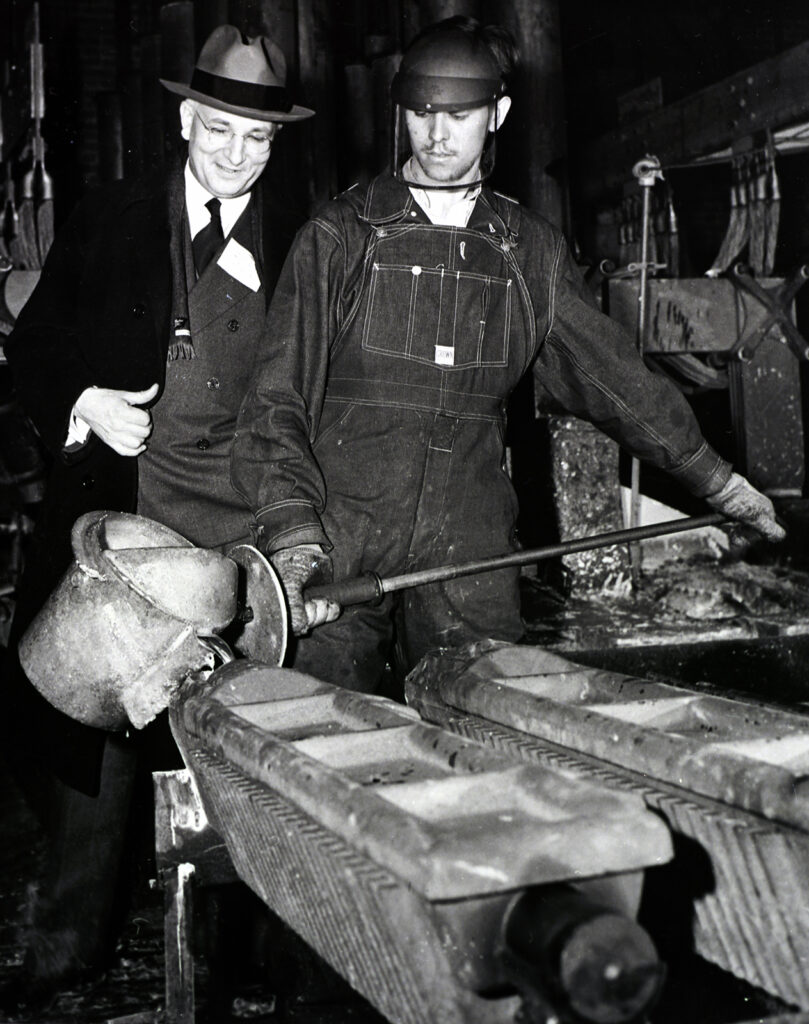
Willard Dow (left) watches Woodrow Rands pour magnesium ingots at a newly opened magnesium plant in Marysville, Michigan, April 1943.
Since brine itself is the residue of ancient oceans, Herbert Dow postulated that if he could extract magnesium from brine, he should also be able to extract it directly from seawater. But with Herbert Dow’s death in 1930, that fantastical idea was left to his son Willard to pursue.
The younger Dow—unfazed by the economic havoc of the Great Depression—dove headlong into the project, setting up a pilot seawater-processing plant at Kure Beach, North Carolina. The plant focused on bromine production, which it sold to the Ethyl Corporation for use in the company’s anti-knock gasoline additive.
At Kure, Dow perfected the process for extracting chemicals directly from the ocean. The pilot plant processed more than six cubic miles of ocean water to produce nearly 2.4 billion pounds of bromine. With World War II looming and the demand for magnesium increasing, creating a magnesium-producing seawater plant became a top priority. Dow soon broke ground on a new seawater plant in Freeport, Texas, and by 1941, it began extracting magnesium from the sea at levels never previously imagined.
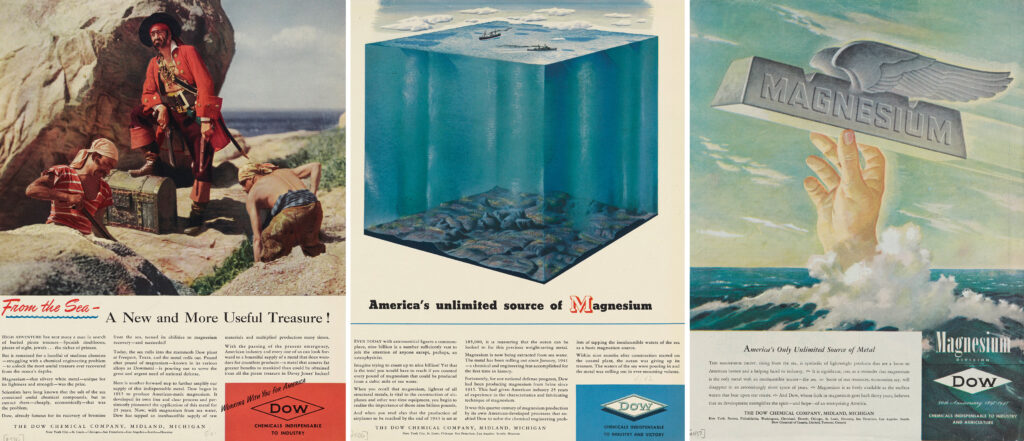
Dow advertisements for ocean-sourced magnesium from 1941, 1942, and 1947, respectively.
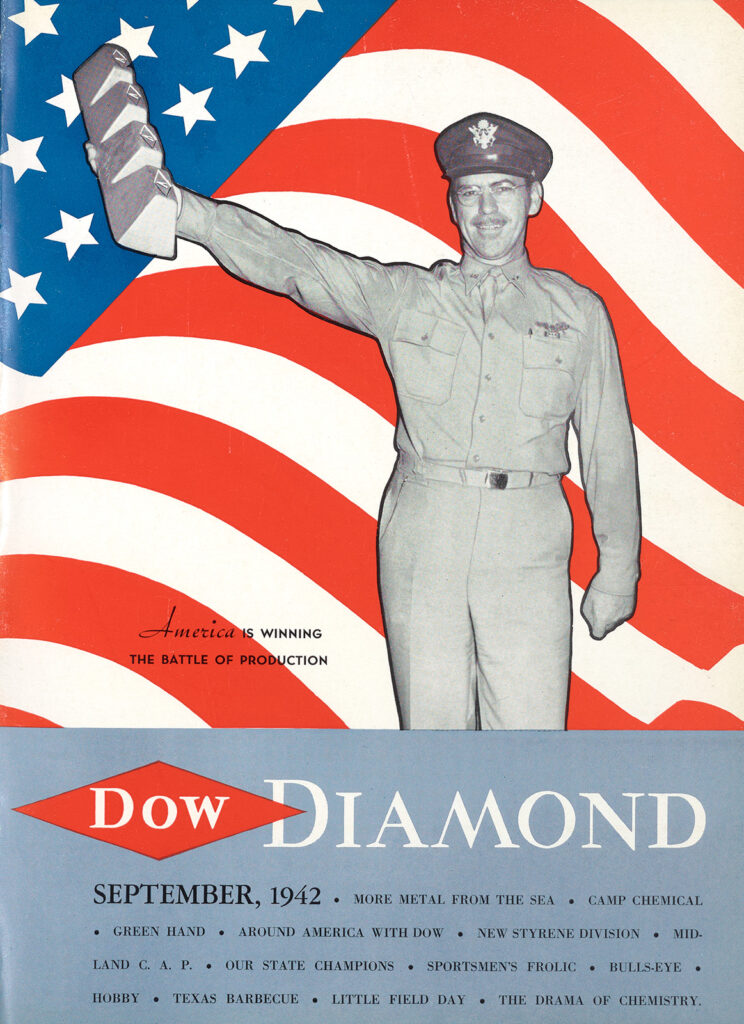
Dow employee and military officer William Cooley holds up a magnesium ingot on the cover of the June 1943 issue of the Dow Diamond, the company’s staff magazine.
The process of extracting metals from the sea involves oyster shells, natural gas, electricity, and a whole lot of water (about 800 tons’ worth for every one ton of magnesium). Heating oyster shells produces calcium oxide, which is mixed with seawater to precipitate magnesium hydroxide. After concentrating the magnesium hydroxide suspension, it is mixed with hydrochloric acid, and the resulting magnesium chloride solution is then evaporated to form a solid cake. This cake is dried and then charged in electrolytic cells, where it is decomposed into chlorine gas and metallic magnesium. The chlorine gas returns to the process by reacting with natural gas to remake hydrochloric acid. The molten magnesium floats to the top of a salt bath, where it is trapped, ladled, and poured into iron ingot molds.
Today seawater is an important source of many minerals, such as sodium, magnesium, sulfur, calcium, and potassium, but in 1939 the idea of recovering a metal from the ocean must have seemed far-fetched to most people and a foolhardy undertaking to Willard Dow’s competitors. But Dow’s gamble proved prescient when the United States entered World War II just months after the Texas plant came online.
In the early days of World War II, the German Luftwaffe dominated the skies over Europe. Its planes flew faster and farther and carried payloads larger than any military experts predicted possible. Studies of German bombers that crashed on British soil revealed that the planes contained a large percentage of magnesium, which greatly reduced the weight of the aircraft and allowed for the increased payloads. These bombs, it turned out, were incendiary weapons also made from magnesium. The Germans had been quietly ramping up magnesium production through the 1930s and by 1938 produced more magnesium than the rest of the world combined.
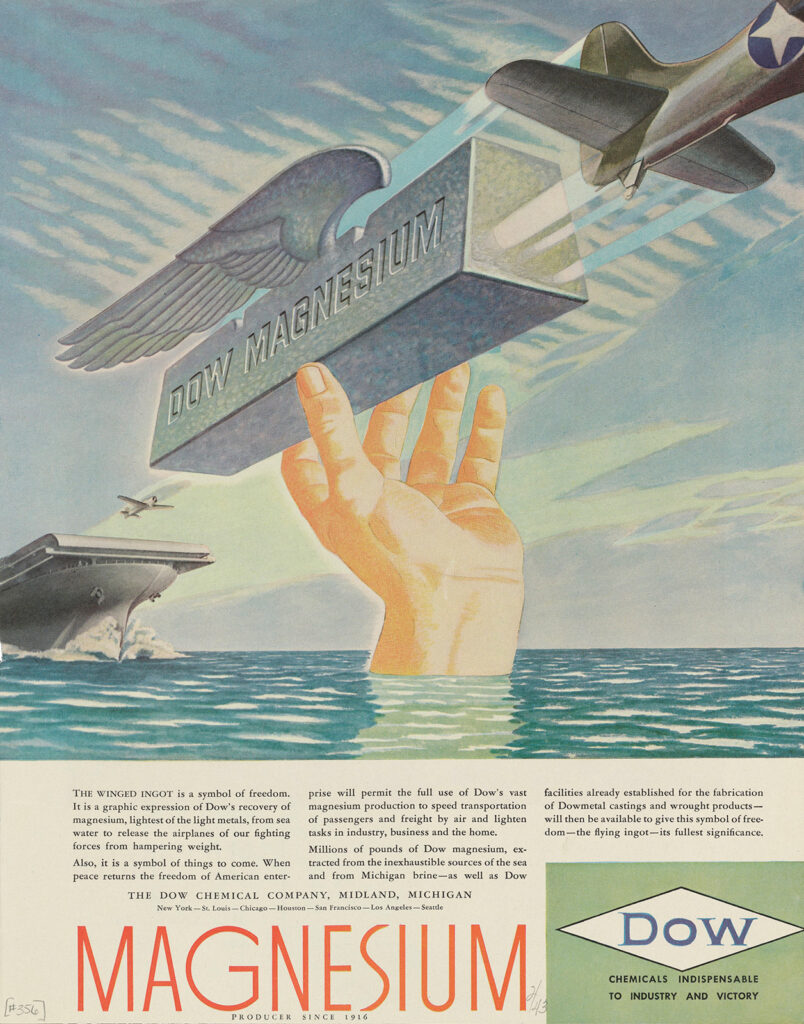
A military-themed ad for Dow magnesium from 1943.
From the earliest days of the Battle of Britain, it became clear that magnesium would play a vital role in the overall war effort. Air power became a significant factor in warfare, and magnesium made the construction of those planes possible. Magnesium was used in the engines, fuselage, and various accessories. Landing wheels in particular contained large amounts of magnesium to take advantage of the metal’s high strength-to-weight ratio and superior shock-absorbing qualities.
In the United States, demand for magnesium grew from six million tons per year to an impossible 800 million tons per year in just a matter of months. Since Dow was the only U.S. manufacturer of magnesium and output at its state-of-the-art seawater plant was capped at about 18 million tons per year, the U.S. government declared magnesium a strategic metal, and all of its production was allocated toward national defense.
The U.S. government also introduced a $400 million program to spur production. By 1943 an additional 14 magnesium plants were up and running, four of them built and operated by Dow.
When military demand for magnesium plummeted after the war, Dow switched gears and sought to incorporate the metal into the postwar explosion of consumer products. Dow marketers hailed magnesium’s ability to reduce the weight of just about every product imaginable—furniture, canoes, vacuum cleaners, wheelbarrows, golf clubs, and lawnmowers, to name just a few.
In the decades that followed, magnesium again was called on to push further into Earth’s atmosphere. It was sent into orbit in July 1962 as part of AT&T’s Telstar 1 communications satellite, which transmitted the first space-relayed telephone calls and television images. Magnesium alloy’s strength, light weight, and heat conductivity made it an ideal choice for the satellite’s outer frame.
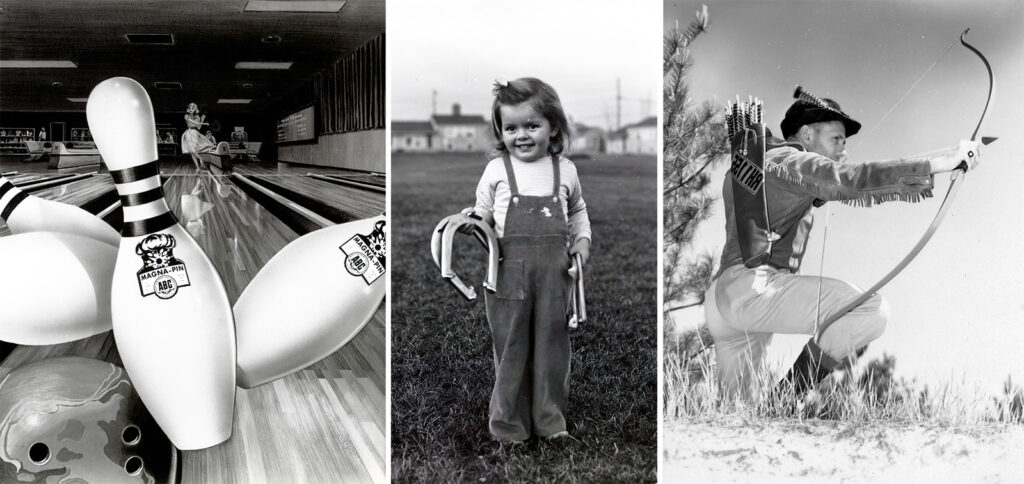
Dow pitched the use of magnesium in many consumer goods after the war including bowling pins (1964), horseshoes (1946), and bows (1947). The magnesium-shell Magna-Pin (left) made it to market as the first artificial pin approved by the American Bowling Congress.
Dow continued to produce magnesium at Freeport until the 1990s. By that time, nearly half the magnesium it produced ended up in aluminum beverage cans. It became clear, however, that profitable magnesium production depended on who had access to the cheapest power. With power costs rising at the Freeport plant and its facilities becoming increasingly antiquated, the writing was on the wall. In 1998 Dow began the shutdown process in Freeport and left the magnesium business entirely.
Today most magnesium is produced in China. It continues to be the third most used structural metal, with its main use as an alloy for aluminum. Because of magnesium’s excellent electrical conductivity and heat dissipation qualities, it is used in many modern-day electronic devices alongside the more buzzworthy rare earth elements, whose limited supplies make national security officials anxious these days. There will always be an ample source of magnesium, however, as long as there are oceans to supply it.